Unlocking the Secrets of Soil: Exploring the Microbiome and Its Applications—Part 1
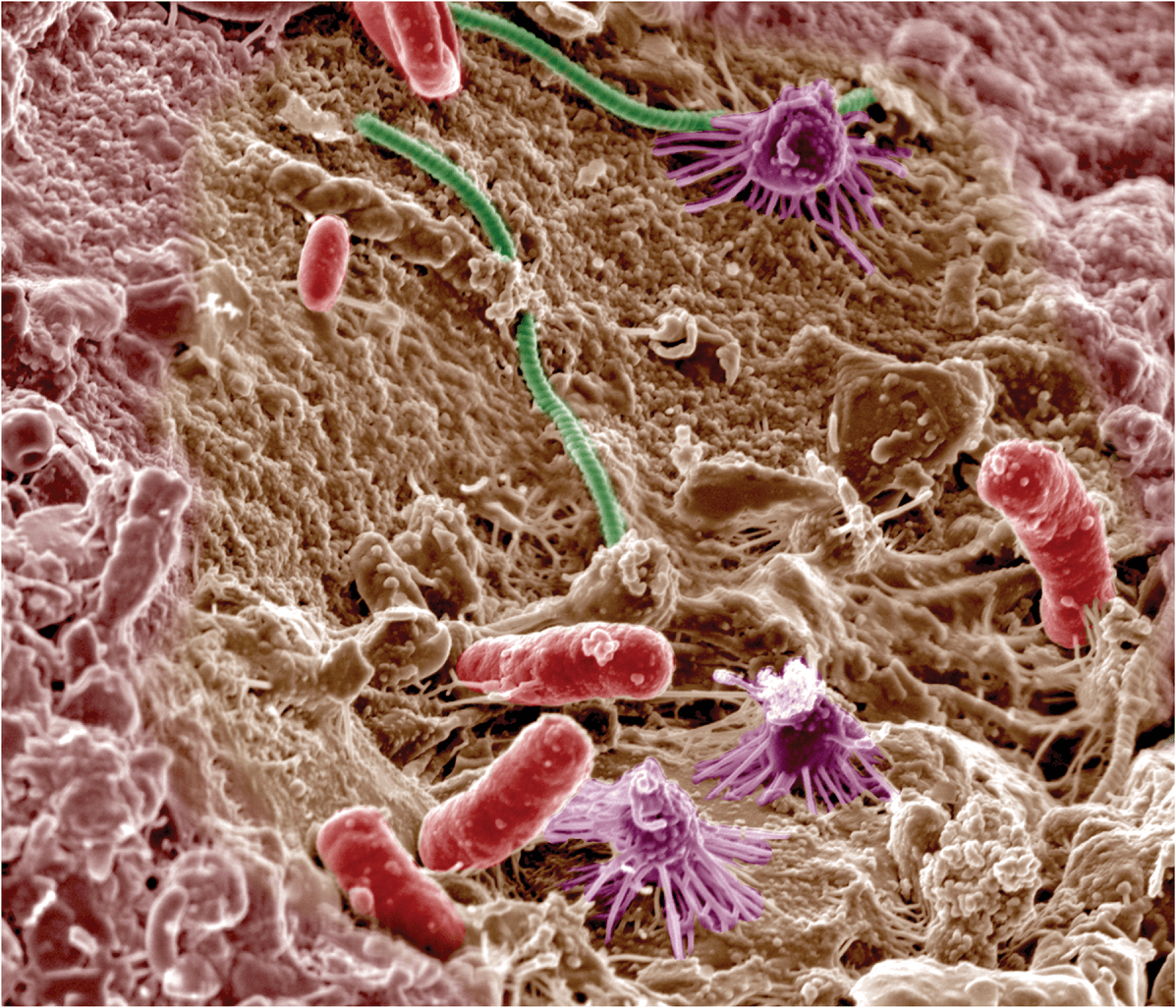
The term “microbiome” refers to a collective assembly of microorganisms within a specific environment, including fungi, bacteria, and viruses, influencing dynamic functions within the system. Microbes play a pivotal role in the production of various food items, wines, and medicinal compounds. Their significance in agriculture and ecology is monumental, which will be explored in this two‐part series. In this first part, we’ll cover microbiome components and applications, the rhizosphere and microbiome interaction, and environmental factors determining the soil–microbe–plant interactions.
View the entire series.
Microbes, the tiny microorganisms ubiquitous in our bodies, food, and environment, wield significant influence over human existence. Throughout human history, we have faced deadly epidemics, such as the bubonic plague in 1500s, which wiped out one‐third of the European population (Glatter & Finkelman, 2021); the Irish potato famine, which caused the death of one million in the Irish population (Kinealy, 1995); the Spanish flu, which resulted in around 50 million deaths in 1918–1920 (CDC, 2019); and the most recent COVID‐19 pandemic, which rapidly engulfed the entire world and caused the death of seven million people (WHO, 2024). These epidemics, driven by viruses, bacteria, or fungi, directly assail human health or indirectly disrupt vital ecosystems supporting life.
Paradoxically, amidst their potential for devastation, microbes also confer invaluable benefits upon us. The presence of microbes in the human gut helps in food digestion, supplies essential metabolites, and bolsters immunity while contributing to the functioning of the brain and whole body systems. Moreover, microbes play a pivotal role in the production of various food items, wines, and medicinal compounds. Their significance in agriculture and ecology is monumental, a topic we shall explore in depth in this two‐part article. Let us commence by delving into the concept of the microbiome and its constituent elements.
The term “microbiome” refers to a collective assembly of microorganisms within a specific environment, including fungi, bacteria, and viruses, influencing dynamic functions within the system. This community shows distinct physicochemical properties, establishing specific ecological niches and forming an interactive and dynamic relationship prone to changes in both time and scale. Microbiomes are categorized based on diverse environments and host organisms, such as human microbiomes, gut microbiomes, soil microbiomes, and root microbiomes. The composition and diversity of microbiomes vary across different systems and environments. For example, the soil microbiome comprises bacteria, fungi, archaea, viruses, small protists, and algae while the human microbiome is predominantly composed of bacteria and fungi with its diversity amounting to only 10% of that found in the soil microbiome (Blum et al., 2019). Furthermore, microbiomes can be commensal, symbiotic, or parasitic, depending on their interactions with the host or other environments.
A gram of soil harbors an impressive 100 million to a billion microorganisms, indicating its richness (Raynaud & Nunan, 2014). The rhizosphere, the region around plant roots, hosts millions and billions of coexisting microbes, including endophytes, mycorrhizae, nitrogen‐fixing and phosphorus‐solubilizing organisms, and saprophytes. These rhizospheric microbes dynamically orchestrate crucial soil processes, including nutrient cycling, soil aggregation, crop nutrient uptake, and plant defense against disease. Furthermore, soil microbes serve a pivotal role in decomposing organic matter, facilitating the release of carbon and nitrogen while harnessing carbon sources from plants via root and mycorrhizal networks.
Microbiome: Components and Applications
In an agroecological context, the microbiome is crucial in fostering plant growth, boosting crop yield, and ameliorating stress resilience. The symbiotic association between microorganisms and plants is pivotal for sustaining agricultural systems. Higher diversity in microbial communities contribute significantly to combatting plant diseases and enhancing crop productivity. Rhizosphere microbes play a vital role in bolstering disease resistance by repelling or outcompeting plant pathogens, forming physical barriers, enhancing nutrient availability, and diminishing resources accessible to pathogens (Li et al., 2021). Additionally, plants release organic compounds through roots, also known as root exudates, which attract and shape microbial dynamics. Approximately 3–30% of the carbon produced from photosynthesis is released as root exudates, thereby regulating microbial activities and influencing plant–microbe interactions (Huang & Osbourn, 2019; Panchal, 2022). Soil microbes, particularly in rhizosphere zones, encompass both saprophytes and symbionts. Saprophytes, including Bacillus, Fusarium, Penicillium, Trichoderma, and Mortierella, thrive by decomposing dead organic matter, thereby enriching carbon and essential nutrients vital to plant nutrition. Symbionts, which include nitrogen‐fixing bacteria, endophytic and epiphytic fungi, and mycorrhizal fungi, form symbiotic relationships with plants, facilitating nutrient acquisition and promoting plant growth (Cahanovitic et al., 2022). All the microbes present in soil are known as soil microbiota.
Let us look at soil microbiota in detail.
Endophytes are endosymbiotic microorganisms that inhabit various plant organs and tissues, serving as significant sources of secondary metabolites, growth‐promoting regulators, and mediators of plant defense signaling. Their presence significantly bolsters plant immunity. They complete their life cycles within plant tissues and provide defense against various pathogens. Notable examples include species like Neotyphodium and Piriformospora, which confer resistance against Fusarium‐ and Sclerotina‐related diseases, respectively. Similarly, Phomopsis liquidambaris elicits resistance against Fusarium oxysporum in peanuts (Zhang et al., 2020). Other common endophytes are Actobacter, Proteobacter, Enterobacter, etc.
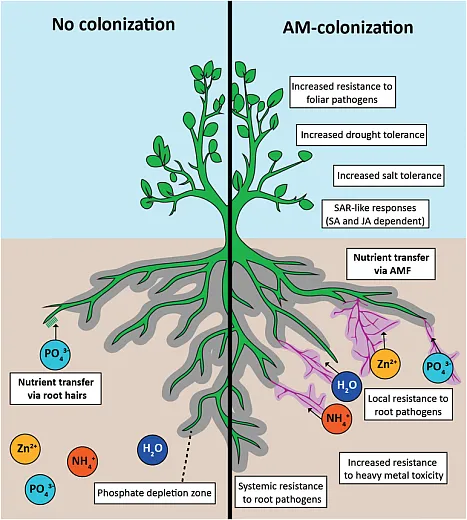
Mycorrhizae are symbiotic fungi that reside within plant roots and require a host to survive and thrive. They obtain their carbon source from host plants and acquire nutrients from the soil, facilitating the indirect absorption of nutrients and water from distant soil regions to the plant roots. There are two types of mycorrhizae: ectomycorrhizae and endomycorrhizae. Ectomycorrhizae, belonging to the Basidiomycetes class, colonize the outer surface of roots, penetrating only the cortex. They are proficient in absorbing phosphate, ammonium, and zinc sources, and their symbiotic relationships are limited to certain plant species. In contrast, endomycorrhizae, also known as arbuscular mycorrhizal fungi, invade plant roots entirely, penetrating cortical cells and establishing hyphal mycelium networks both internally and externally. These fungi, classified as phycomycetes, are adept at absorbing phosphate, zinc, nitrate, water, and various other nutrients.
Mycorrhizae also play a multifaceted role in plant defense against soil‐borne pathogens while simultaneously facilitating nutrient and water uptake. They also assist plants during different harsh environmental conditions such as drought, salinity, high temperatures, or heavy metal contamination by providing nutrients and water to stressed roots from distant zones where plant roots can’t reach. They are present in a wide range of plants, from agronomic crops to different grasses, shrubs, and trees. Many agronomic crops like Solanacea, Fabaceae, and Allium crops receive 42–95% of their phosphorus needs from mycorrhizae. Variability in phosphorus acquisition among different genotypes is attributed to variations in mycorrhizal colonization levels (Elbon & Whalen, 2015). Mycorrhizae can provide up to 71% of ammonium resources to a maize crop (Tanaka & Yano, 2005).
Some of the free‐living bacteria and fungi like Pseudomonas, Bacillus, Arthobacter, Acinetobacter, Laeclercia, and fungi like Penicillium, Aspergillus and Trichoderma are also considered as beneficial microbes as they can fix nitrogen and solubilize many chelated minerals into accessible forms. These microbes serve as catalysts for nutrient recycling and play an indispensable role in soil decompositions, aggregations, and nutrient provision. Their concerted efforts can lead to a potential yield increase of up to 70%.
Overall, microbial‐mediated decomposition plays a crucial role in balancing the carbon and nitrogen equilibrium in the soil, thereby contributing to soil carbon storage. Approximately 80% of the 2500 gigatonnes of carbon stored in soil is directly regulated by soil microbes, underscoring their paramount importance in the global carbon pool (Gougoulias et al., 2014). Furthermore, soil microbes actively contribute to soil formation by enhancing soil aggregation, a process critical for enhancing soil fertility, porosity, water‐holding capacity, and promoting seed germination and crop yield.
Rhizosphere and Microbiome Interaction
Plants, particularly their roots, forge intricate connections with microbes through the interface between the soil and roots known as rhizosphere. Rhizosphere is rich in root exudates, thereby holding higher diversity and composition of microbial communities, which thrive on carbon and metabolites released from exudates. Root exudates, comprising terpenes, flavonoids, phytohormones, amino acids, lignin, and proteins, serve as crucial components of microbe–plant interactions. For example, terpenes, essential secondary metabolites, attract beneficial microbes such as mycorrhiza and rhizobacteria, and flavonoids released from roots act as a precursor for establishing symbiotic relationships between plants and rhizobacteria (Kumar et al., 2023). Simultaneously, the presence of microbes in the rhizosphere can pose challenges to plant defense mechanisms. In response, plants release phytohormones, including salicylic acid, ethylene, jasmonic acid, auxin, and abscisic acid, to coordinate diverse defense mechanisms essential for safeguarding plant health and immunity against microbial presence.
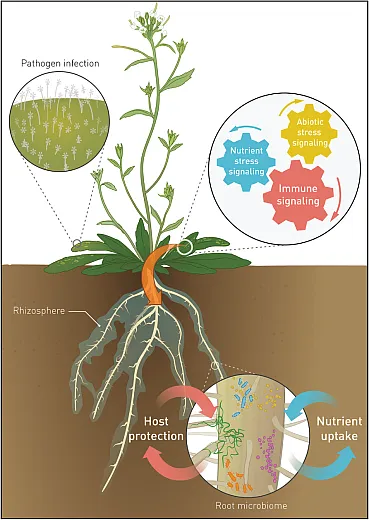
Moreover, microbes possess a remarkable ability to synthesize plant hormones, including ethylene, auxins, cytokinin, abscisic acid, and gibberellins, along with their precursors, thereby influencing plant growth and hormonal signaling. The interplay among plant immunity, the release of different secondary metabolites and root exudates, and extracellular compounds by microbes, profoundly shapes microbial associations, compositions, and diversity. Additionally, microbes produce quorum‐sensing compounds, including extracellular signaling molecules like acyl homoserine lactone, oligopeptides, and gamma butyrolactones, which are essential for microbial interspecies communication (Chagas et al., 2018). Certain rhizobium bacteria secrete extracellular substances like effector proteins and exopolysaccharides to facilitate interactions between microbes and their environment as well as between different microbial species (Costa et al., 2021). Microbial biofilm is another significant component of the microbiome, providing protection and support for microbial survival and activity in diverse environmental conditions.
Thus, the rhizosphere region, as a hotspot with an abundance of root exudates, can attract varied microbes, subsequently improving the soil microbial communities and diversities. The composition of root exudates plays an important role in shaping rhizosphere microbial communities through selective enrichment of microbes capable of utilizing exuded compounds. The types and quantities of organic molecules exuded, along with microbial metabolic versatility, determine whether epiphytic, endophytic, or free‐living rhizosphere communities develop upon plant contact. Rhizosphere metabolites modulate relationships between plants and associated microbes that span pathogenic, neutral, and mutualistic. These complex and variable plant–microbe associations largely determine host plant survival and productivity. However, plant microbiome diversity and assembly rules remain poorly understood. Elucidating modulatory strategies plants utilize to optimize beneficial associations requires deeper characterization of rhizosphere community assembly principles and species interactions. This knowledge promises to enable management leveraging endogenous microbiomes for enhanced plant growth and stress resilience in agricultural systems.
Environmental Factors Determining the Soil–Microbe–Plants Interactions
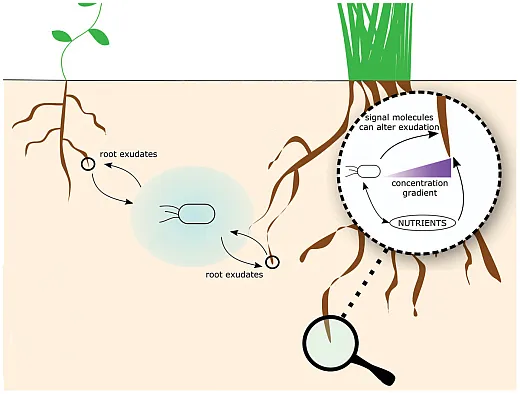
Understanding the intricate interplays among soil, microbes, and plants is fundamental to ecosystem dynamics and sustainability. These interactions are heavily influenced by a myriad of environmental factors. These factors encompass a broad range of physical, chemical, biological, and climate variables that collectively share the structure and functions of soil microbial communities and their interactions with plants. Understanding these factors is essential in elucidating the complex microbial ecology and remodeling them for agriculture and ecological advances. We will explore some of the major environmental factors that shape the soil–plant microbe interactions.
Soil Characteristics
Soil characteristics such as soil texture, structure, pH, temperature, moisture content, and nutrient availability shape the distribution and diversity of microbial communities and their activities (Girvan et al., 2003). These factors influence the microbial‐mediated formation of soil aggregates in the feedback loop (Tisdall, 2020). For example, a study reported that soil moisture content exerts a higher impact on the structure of the microbial community and their metabolism and nutrient cycling ability (Singh et al., 2009). Soil pH not only directly influences nutrient availability but also serves as a crucial limiting factor for microbial soil communities (Zhalnina et al., 2015). For instance, soil pH highly determines the availability of phosphorus in soil, which in turn affects the growth of arbuscular mycorrhizal fungi and their metabolic activities. Further, soil pH influences the microbial community compositions with certain microbial species thriving in acidic soils while others prefer alkaline conditions. The abundance of mycorrhiza is directly related to crop health and productivity. Increasing atmospheric temperature can also heat up the soil; this modifies the structure of the rhizosphere microbiome and impacts the microbial activity, especially the conversion of organic matter to inorganic nutrients (Santoy et al., 2017).
Climate and Weather
Several studies have reported that elevated carbon dioxide reduces the microbial diversity and nitrogen fixation (Yang et al., 2019) while another study reported that elevated carbon dioxide can increase the mycorrhizal abundance and activity (Dhillion et al., 1995). Precipitation regimes also affect the soil microbial growth and metabolic activity, thus affecting diversity and composition, and altering the organic matter decomposition and nutrient recycling (Likar et al., 2013). Extreme events like droughts, floods, and heat waves can disrupt the soil microbial community and alter the plant microbial association, affecting plant productivity and ecosystem functioning. Overall, climate change exerts a significant impact in plant microbial interactions.
Land Use and Management Practices
Intensive agricultural practices, including excessive use of fertilizers and pesticides, monocropping, and deep tillage greatly disturb soil microbial activity, composition, and diversity. Several studies have shown that monocultures reduce soil microbial diversity and increase host‐specific pathogens, deep tillage alters the microbial composition by altering soil microclimate, high nitrogen deposition leads to soil acidification and reduced soil microbial biomass, and pesticides create a toxic environment for the beneficial microbes, thus impacting the overall soil microbial activity and compositions (Yang et al., 2021).
Plant Species and Diversity
Plant species and diversity exert profound influence on soil microbial communities and their interactions with plants. Different plant species have different levels of root exudates and their compositions, thus shaping the microbial community structure and functions in the rhizosphere. High plant diversity increases rhizosphere carbon inputs into the microbial community, which results in increasing microbial activity, microbial biomass, microbial respiration and carbon storage (Lange et al., 2015; Chen et al., 2019). Recent studies in adopting cover crops in agricultural practices have shown significant improvement in soil microbial diversity and functional groups, thus enhancing plant–microbe interaction and plant productivity (Vukicevich et al., 2016; Finney et al., 2017).
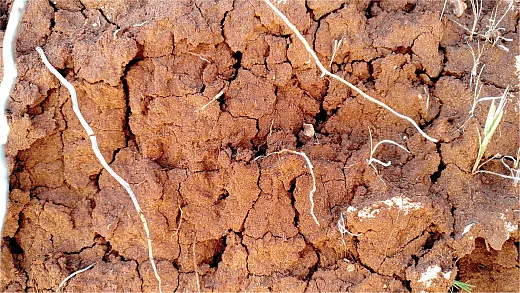
References
Blum, W.E., Zechmeister‐Boltenstern, S., & Keiblinger, K.M. (2019). Does soil contribute to the human gut microbiome? Microorganisms, 7(9), 287.
CDC. (2019). The discovery and reconstruction of the 1918 pandemic virus. Centers for Disease Control and Prevention.
Chagas, F.O., de Cassia Pessotti, R., Caraballo‐Rodriguez, A.M., & Pupo, M.T. (2018). Chemical signaling involved in plant–microbe interactions. Chemical Society Reviews, 47(5), 1652–1704.
Chen, C., Chen, H.Y., Chen, X., & Huang, Z. (2019). Meta‐analysis shows positive effects of plant diversity on microbial biomass and respiration. Nature communications, 10(1), 1332.
Costa, S.R., Ng, J.L.P., & Mathesius, U. (2021). Interaction of symbiotic rhizobia and parasitic root‐knot nematodes in legume roots: from molecular regulation to field application. Molecular Plant‐Microbe Interactions, 34(5), 470–490.
Dhillion, S.S., Roy, J., & Abrams, M. (1995). Assessing the impact of elevated CO 2 on soil microbial activity in a Mediterranean model ecosystem. Plant and Soil, 187, 333–342.
Elbon, A., & Whalen, J.K. (2015). Phosphorus supply to vegetable crops from arbuscular mycorrhizal fungi: a review. Biological Agriculture & Horticulture, 31(2), 73–90.
Finney, D.M., Buyer, J.S., & Kaye, J.P. (2017). Living cover crops have immediate impacts on soil microbial community structure and function. Journal of Soil and Water Conservation, 72(4), 361–373.
Girvan, M.S., Bullimore, J., Pretty, J.N., Osborn, A. M., & Ball, A. S. (2003). Soil type is the primary determinant of the composition of the total and active bacterial communities in arable soils. Applied and Environmental Microbiology, 69(3), 1800–1809.
Glatter, K.A., & Finkelman, P. (2021). History of the plague: An ancient pandemic for the age of COVID‐19. The American journal of medicine, 134(2), 176–181.
Gougoulias, C., Clark, J.M., & Shaw, L.J. (2014). The role of soil microbes in the global carbon cycle: tracking the below‐ground microbial processing of plant‐derived carbon for manipulating carbon dynamics in agricultural systems. Journal of the Science of Food and Agriculture, 94(12), 2362–2371.
Huang, A.C., & Osbourn, A. (2019). Plant terpenes that mediate below‐ground interactions: prospects for bioengineering terpenoids for plant protection. Pest Management Science, 75(9), 2368–2377.
Kinealy, C. (1995). This great calamity: the Irish famine, 1848–1852. UNKNO.
Kumar, G.A., Kumar, S., Bhardwaj, R., Swapnil, P., Meena, M., Seth, C. S., & Yadav, A. (2023). Recent advancements in multifaceted roles of flavonoids in plant–rhizomicrobiome interactions. Frontiers in Plant Science, 14.
Lange, M., Eisenhauer, N., Sierra, C.A., Bessler, H., Engels, C., Griffiths, R. I., … & Gleixner, G. (2015). Plant diversity increases soil microbial activity and soil carbon storage. Nature Communications, 6(1), 6707.
Li, J., Wang, C., Liang, W., & Liu, S. (2021). Rhizosphere microbiome: The emerging barrier in plant‐pathogen interactions. Frontiers in Microbiology, 12, 772420.
Raynaud, X., & Nunan, N. (2014). Spatial ecology of bacteria at the microscale in soil. PloS One, 9(1), e87217.
Singh, B.K., Dawson, L.A., Macdonald, C.A., & Buckland, S.M. (2009). Impact of biotic and abiotic interaction on soil microbial communities and functions: A field study. Applied Soil Ecology, 41(3), 239–248.
Tanaka, Y., & Yano, K. (2005). Nitrogen delivery to maize via mycorrhizal hyphae depends on the form of N supplied. Plant, Cell & Environment, 28(10), 1247–1254.
Tisdall, J.M. (2020). Formation of soil aggregates and accumulation of soil organic matter. In Structure and organic matter storage in agricultural soils (pp. 57–96). CRC Press.
Vukicevich, E., Lowery, T., Bowen, P., Úrbez‐Torres, J.R., & Hart, M. (2016). Cover crops to increase soil microbial diversity and mitigate decline in perennial agriculture. A review. Agronomy for Sustainable Development, 36, 1–14.
Yang, S., Zheng, Q., Yuan, M., Shi, Z., Chiariello, N.R., Docherty, K.M., … & Yang, Y. (2019). Long‐term elevated CO2 shifts composition of soil microbial communities in a Californian annual grassland, reducing growth and N utilization potentials. Science of the Total Environment, 652, 1474–1481.
Yang, T., Lupwayi, N., Marc, S.A., Siddique, K.H., & Bainard, L.D. (2021). Anthropogenic drivers of soil microbial communities and impacts on soil biological functions in agroecosystems. Global Ecology and Conservation, 27, e01521.
Zhalnina, K., Dias, R., de Quadros, P.D., Davis‐Richardson, A., Camargo, F.A., Clark, I.M., … & Triplett, E.W. (2015). Soil pH determines microbial diversity and composition in the park grass experiment. Microbial Ecology, 69, 395–406.
Zhang, F.M., He, W., Wu, C.Y., Sun, K., Zhang, W., & Dai, C.C. (2020). Phomopsis liquidambaris inoculation induces resistance in peanut to leaf spot and root rot. BioControl, 65, 475–488.
Text © . The authors. CC BY-NC-ND 4.0. Except where otherwise noted, images are subject to copyright. Any reuse without express permission from the copyright owner is prohibited.