Fostering Microbial Activity and Diversity in Agricultural Systems
Adopting Better Management Practices and Strategies: Part 3
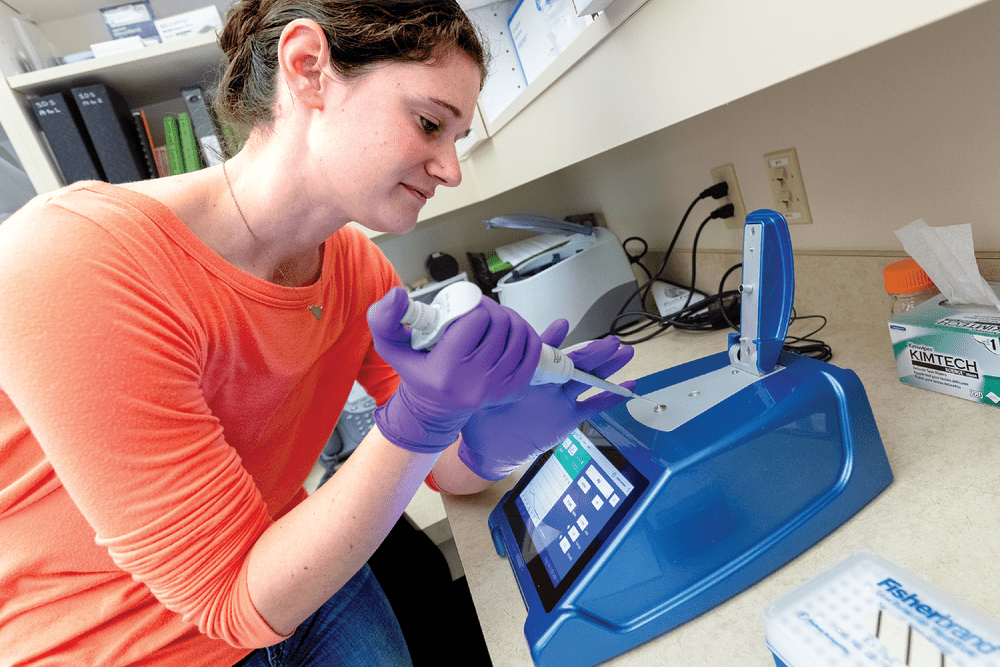
This article is the third in a three‐part series on fostering microbial activity and diversity through better management practices and strategies. Part 3 will discuss monitoring and quantification of microbial diversity, challenges for adopting sustainable practices, government policy, and scaling microbial diversity from smaller to larger agricultural systems.
Monitoring and Quantification of Microbial Diversity and Its Impacts
Soil microbial diversity is a black box and requires more intensive study to understand the effects of various strategies on ecosystem functions. The monitoring and assessment of soil microbial activity, abundance, and diversity will help us predict the associated soil properties and processes for assessing the soil health and impacts on crop production. Various methods are employed to monitor and quantify the soil microbial communities. Most of these methods need sophisticated tools and controlled environments, which may not be available for farmers but are definitely available at local university, government, and privately run labs.
Plate and direct counts involve culturing microorganisms on media and counting them under a microscope, primarily detecting culturable microbes (Bakken, 1997). Phospholipid fatty acid analysis (PLFA) extracts and analyzes phospholipids from microbial cells, differentiating bacterial and fungal groups. Real‐time polymerase chain reaction (PCR) measures DNA amplification to quantify the microbial community quantitatively but lacks qualitative insights. Gene sequencing assesses alpha and beta diversity, offering a qualitative understanding of microbial communities resulting from different management practices. Enzymatic activity assays are commonly used to understand soil microbial roles in carbon and nutrient cycles by breaking down compounds into simpler forms. Soil enzymes, mainly extracellular, play a crucial role in decomposing organic compounds with hydrolytic and oxidative enzymes involved in carbon, nitrogen, and phosphorus cycling. Another method, the BIOLOG EcoPlate, assesses community‐level physiological profiling (CLPP) by providing 31 carbon substrates and measuring microbial metabolic potential through color development and optical density at 590 nm (Garland & Mills, 1991). These techniques offer valuable insights into soil microbial functions and their contributions to ecosystem processes.
Researchers are increasingly concerned about the disparity between soil microbial exploration in laboratory settings and real‐world field conditions. There is a pressing need for a farmer‐friendly and comprehensive approach for rapid on‐farm assessment of soil microbial diversity, which could facilitate a more accurate correlation between soil microbial diversity and soil functions as well as ecosystem services.
Challenges of Adopting Sustainable Practices to Foster Soil Microbial Health
There are several challenges and limitations in advancing microbial diversity.
Financial Burden
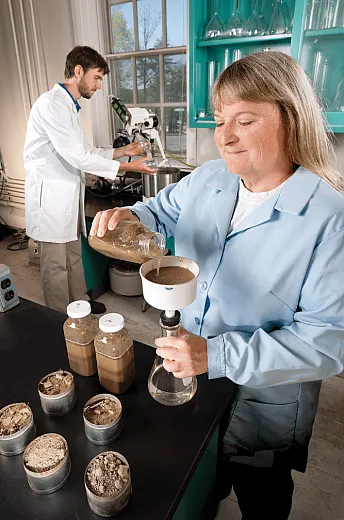
Money and resources are always significant criteria for farmers and other agricultural managers throughout the growing season. To test for microbial populations, farmers typically send soil samples to commercial or extension laboratories for analysis; however, testing can be costly depending on the materials/technology necessary for analysis and the number of samples. Microbial inoculants for in situ enhancement of microbial populations may also be expensive depending on the acreage and the frequency of application needed for desired influence in the soil microbiome. Adopting cultural practices like conservation tillage, crop rotation, intercropping, and cover crops will build up microbial activity and diversity in the long run; however, they could pose a financial burden to the farmers in the short term. A recent study (Pathak et al., 2024) has shown that farmers are likely to discontinue cover cropping and conservation tillage when there is no government funding available, which shows that there is a need to support farmers financially to achieve the goal of a sustainable future through microbial farming.
Agronomic Challenges
Agronomic management practices play an immense role in enhancing microbial diversity and activity. Shifting agricultural management from a conventional system to management practices such as cover cropping, crop rotation, and/or conservation tillage can beneficially magnify the microbial population. Shifting between management practices can be difficult, especially considering the benefits may appear gradually over the long term. Farmers may find it difficult to continue practicing management alternatives when they have a slew of immediate problems such as increased weed pressures with conservation tillage (Kumar et al., 2020).
Another challenge in agronomic systems is the effect of pesticides on native microbial populations. Depending on the type of pesticide applied and the growing crop, the application of pesticides may alter microbial populations and/or affect their niche roles within the soil–plant ecosystem (Yu et al., 2023) With the additional influence of increased weed pressures from sustainable management practices, increasing the frequency of pesticide application leads to indirect and direct effects on the microbial population (Lo, 2010; Yu et al., 2023).
Human Health Risk of Organic Fertilizers
As discussed earlier, organic fertilizers are a fantastic alternative to inorganic fertilizers for stimulating microbial activity in the soil microbiome; however, depending on the source of the organic fertilizer (animal waste, compost, or biosolids), contaminants of concern such as heavy metals, pharmaceuticals, and hormones may be entering the soil and be available for plant uptake or may enter water resources (Goss et al., 2013; Urra et al., 2019). Not all organic fertilizers have these contaminants of concern as it entirely depends on the source and the treatment of these organic fertilizers in preparation for agricultural application; it is important to recognize the potential of introducing these contaminants of concern to our food and water resources.
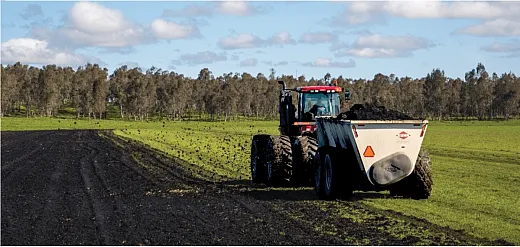
Education
Finally, the last and probably most important challenge is educating agricultural managers, farmers, and home gardeners on the essentiality of a diverse and active soil microbiome in crop production. Microbes tend to have a bad reputation with the general public, mainly known to be harmful to human health and causing disease. While this is not inherently incorrect, it is still a misperception as there are a multitude of beneficial microbes that are necessary for everyday life. It is important to improve microbial literacy so that agricultural managers and home gardeners can make informed decisions for their agroecosystems (Bloom et al., 2024).
Government Policy: Regulations and Interventions
In recent decades, there's been a notable surge in microbiome research, particularly in agricultural contexts aimed at bolstering crop production amidst climate change. While soil management is vital for climate‐resilient agriculture, focusing on soil microbiome diversity is crucial for sustainability. Understanding these interactions is key to optimizing microbe benefits. Thus, a strong government policy is needed to effectively incorporate the policy of diversifying and harnessing the microbiome's potential. The soil microbiome has been further integrated to the One Health initiative where microbes are recognized as integral components of human animal and ecological health. This underscores the importance of soil microbiome research and development as a cornerstone of achieving One Health (Banerjee & Van Der Heijden, 2023).
There have been many initiatives from the U.S. government in promoting the study of microbiomes in a broader perspective and exploring their potential. In 2016, the U.S. government launched the National Microbiome Initiative (NMI), investing more than $121 million to advance cross‐ecosystem microbiome studies, which profoundly impact human health, food security, and ecosystem functioning (White House Office of Science and Technology Policy, 2016). Similarly, the Food and Drug Administration (FDA) has implemented regulations for monitoring the impacts of FDA‐regulated products on microbiomes. The U.S. government's 2023 farm bill prioritizes soil carbon capture and storage, emphasizing strategies to enhance microbial activity and diversity in agricultural systems, underlining the importance of policymaking in implementing microbiome‐based strategies while preserving agricultural soils (Tao et al., 2023). The bill incentivizes the use of biologicals, which can improve root traits, nutrient cycling, and carbon sequestration, ultimately reducing greenhouse gas emissions and increasing return on investment. Through the Inflation Reduction Act (IRA), the U.S. government allocated $20 billion for conservation and climate‐smart agriculture and $300 million for actions addressing soil carbon improvement, nitrogen loss reduction, and mitigation of agriculture‐related greenhouse gasses and microbial biodiversity. Furthermore, the National Institute of Food and Agriculture (NIFA) under USDA has prioritized research funding towards agricultural microbiomes, aiming to enhance productivity, sustainability, food safety, and carbon sequestration in agricultural systems while addressing knowledge gaps in agricultural microbes and microbiome functions across different scales of agricultural ecosystems through innovative projects (NIFA, 2023). Going further, the government should develop a pricing formula to reward farmers or farms that sequester more carbon, thereby encouraging them. Additionally, to achieve the goal of net zero emissions by 2050 in the U.S., we must reduce emissions from agriculture where microbiomes will play a crucial role (Kerry & McCarthy, 2021).
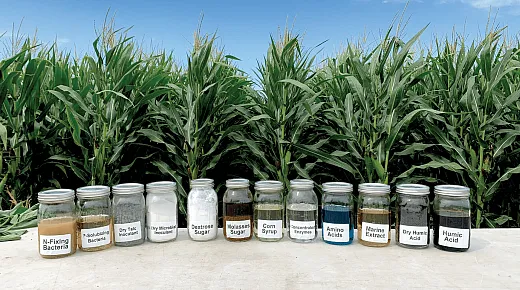
Scaling Microbial Diversity From Smaller to Larger Agricultural Systems
Microbial communities and their diversity are crucial for ecosystem resilience (Shade et al., 2012). Adopting agroecological principles like maximizing biodiversity and promoting soil cover enhances soil organic matter and microbial activity and diversity, leading to improved carbon sequestration, water infiltration, wildlife habitats, and profitability (Prommer et al., 2020). Microbes play a vital role in soil health and crop growth while providing ecosystem services to combat climate change (McBratney et al., 2014). Scaling up practices to promote beneficial soil microbes globally requires continuous improvement in farming practices and support for farmers to adopt sustainable methods, ensuring soil health, carbon sequestration, and food security.
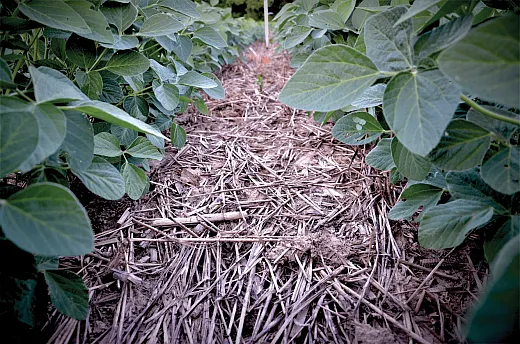
Conventional farming persists due to the pressure of global food demand, yet strategies can mitigate its environmental impact. Implementing crop rotation, intercropping, and reduced synthetic inputs in conventional farming enhances microbial diversity and reduces soil disturbance (Labouyrie et al., 2023). Organic farming fosters richer microbial diversity than conventional methods, benefiting from practices like crop rotation, organic inputs, and increased plant diversity (Hartmann et al., 2015; Lori et al., 2017). Scaling up organic farming and adopting its standardized practices can lead to a sustainable future. Urban gardening, though small in scale, contributes significantly to food security and sustainability through practices like composting, mixed cropping, and animal farming (Bonanomi et al., 2016; Lupatini et al., 2017). Permaculture and conservation agriculture emphasize holistic approaches, promoting soil health and microbial diversity through reduced inputs and whole‐system management (Symanczik et al., 2017; De Tombeur et al., 2018; Jia et al., 2022). These practices offer promising pathways toward sustainable agriculture and climate resilience.
“…there can be no life without soil and no soil without life; they have evolved together.”
—Charles Edwin Kellogg
Conclusion and Future Directions
At this pivotal moment, optimism surrounds the evolving understanding of the role of microbes in agroecosystems, yet caution is warranted amidst increasingly complex global challenges. Embracing the task of scaling up microbial knowledge to a global level can lead to a more sustainable and resilient agricultural future. A thriving soil microbiome forms the bedrock of sustainable agriculture, driving ecological functions and contributing to nutrient cycling, soil structure maintenance, plant growth, and carbon capture. Nurturing microbial activity and diversity is vital for preserving soil fertility, mitigating climate change impacts, and ensuring long‐term food security. While promising practices like reduced tillage and regenerative agriculture show potential, the future lies in harnessing plant–microbe partnerships and leveraging technologies like gene editing to engineer beneficial microbial strains. Robust policy support and advocacy efforts are needed for widespread adoption of ecological practices to enhance the soil microbiome. Farmers, policymakers, researchers, and the public must collaborate to develop comprehensive soil health strategies, implement evidence‐based practices, and quantify the benefits of agroecological approaches. Through collective efforts and a commitment to soil stewardship, we can unlock the soil microbiome's full potential, restoring degraded soils, enhancing food security, and mitigating climate change for a sustainable future.
References
Bakken, L. R. (1997). Culturable and non‐culturable bacteria in soil. In J.D. van Elsas, J.T. Trevor & E.M.H. Wellington (Eds.), Modern soil microbiology (pp. 47–61). Marcel Dekker.
Banerjee, S., & Van Der Heijden, M. G. (2023). Soil microbiomes and one health. Nature Reviews Microbiology, 21(1), 6–20.
Bloom, E. H., Atallah, S. S., & Casteel, C. L. (2024). Motivating organic farmers to adopt practices that support the pest‐suppressive microbiome relies on understanding their beliefs. Renewable Agriculture and Food Systems, 39, e8.
Bonanomi, G., De Filippis, F., Cesarano, G., La Storia, A., Ercolini, D., & Scala, F. (2016). Organic farming induces changes in soil microbiota that affect agro‐ecosystem functions. Soil Biology and Biochemistry, 103, 327–336.
De Tombeur, F., Sohy, V., Chenu, C., Colinet, G., & Cornelis, J. T. (2018). Effects of permaculture practices on soil physicochemical properties and organic matter distribution in aggregates: A case study of the bec‐hellouin farm (France). Frontiers in Environmental Science, 6, 116.
Garland, J. L., & Mills, A. L. (1991). Classification and characterization of heterotrophic microbial communities on the basis of patterns of community‐level sole‐carbon‐source utilization. Applied and Environmental Microbiology, 57(8), 2351–2359.
Goss, M. J., Tubeileh, A., & Goorahoo, D. (2013). A review of the use of organic amendments and the risk to human health. Advances in Agronomy, 120, 275–379.
Hartmann, M., Frey, B., Mayer, J., Mäder, P., & Widmer, F. (2015). Distinct soil microbial diversity under long‐term organic and conventional farming. The ISME journal, 9(5), 1177–1194.
Jia, L., Wang, Z., Ji, L., De Neve, S., Struik, P. C., Yao, Y., … & Jin, K. (2022). Keystone microbiome in the rhizosphere soil reveals the effect of long‐term conservation tillage on crop growth in the Chinese Loess Plateau. Plant and Soil, 473(1), 457–472.
Kerry, J., & McCarthy, G. (2021). The long‐term strategy of the United States, pathways to net‐zero greenhouse gas emissions by 2050. The United States Department of State and the United States Executive Office of the President.
Kumar, V., Mahajan, G., Dahiya, S., & Chauhan, B. S. (2020). Challenges and opportunities for weed management in no‐till farming systems. In Yash P. Dang, Ram C. Dalal & Neal W. Menzies (Eds.), No‐till farming systems for sustainable agriculture: challenges and opportunities (pp. 107–125). Springer.
Labouyrie, M., Ballabio, C., Romero, F., Panagos, P., Jones, A., Schmid, M. W., … & Orgiazzi, A. (2023). Patterns in soil microbial diversity across Europe. Nature Communications, 14(1), 3311.
Lo, C. C. (2010). Effect of pesticides on soil microbial community. Journal of Environmental Science and Health Part B, 45(5), 348–359.
Lori, M., Symnaczik, S., Mäder, P., De Deyn, G., & Gattinger, A. (2017). Organic farming enhances soil microbial abundance and activity—A meta‐analysis and meta‐regression. PloS One, 12(7), e0180442.
Lupatini, M., Korthals, G. W., De Hollander, M., Janssens, T. K., & Kuramae, E. E. (2017). Soil microbiome is more heterogeneous in organic than in conventional farming system. Frontiers in Microbiology, 7, 2064.
McBratney, A., Field, D. J., & Koch, A. (2014). The dimensions of soil security. Geoderma, 213, 203–213.
Pathak, S., Wang, H., Tran, D. Q., & Adusumilli, N. C. (2024). Persistence and disadoption of sustainable agricultural practices in the Mississippi Delta region. Agronomy Journal, 116(2), 765–776.
Prommer, J., Walker, T. W., Wanek, W., Braun, J., Zezula, D., Hu, Y., … & Richter, A. (2020). Increased microbial growth, biomass, and turnover drive soil organic carbon accumulation at higher plant diversity. Global Change Biology, 26(2), 669–681.
Shade, A., Peter, H., Allison, S. D., Baho, D. L., Berga, M., Bürgmann, H., … & Handelsman, J. (2012). Fundamentals of microbial community resistance and resilience. Frontiers in microbiology, 3, 417.
Symanczik, S., Gisler, M., Thonar, C., Schlaeppi, K., Van der Heijden, M., Kahmen, A., … & Mäder, P. (2017). Application of mycorrhiza and soil from a permaculture system improved phosphorus acquisition in naranjilla. Frontiers in Plant Science, 8, 1263.
Tao, F., Huang, Y., Hungate, B. A., Manzoni, S., Frey, S. D., Schmidt, M. W., … & Luo, Y. (2023). Microbial carbon use efficiency promotes global soil carbon storage. Nature, 618(7967), 981–985.
Urra, J., Alkorta, I., & Garbisu, C. (2019). Potential benefits and risks for soil health derived from the use of organic amendments in agriculture. Agronomy, 9(9), 542.
White House Office of Science and Technology Policy. (2016). Fact sheet: Announcing the National Microbiome Initiative.
Yu, Z., Lu, T., & Qian, H. (2023). Pesticide interference and additional effects on plant microbiomes. Science of The Total Environment, 888, 164149.
Text © . The authors. CC BY-NC-ND 4.0. Except where otherwise noted, images are subject to copyright. Any reuse without express permission from the copyright owner is prohibited.