Fostering Microbial Activity and Diversity in Agricultural Systems
Adopting Better Management Practices and Strategies: Part 1

Microbes are tiny organisms that are present in our bodies, food, and environment. They are bacteria, fungi, viruses, protozoans, etc. Microbes show distinct functional features in different hosts and environments. This gives rise to the term “microbiome,” which is defined as the group of microorganisms residing in a particular system and showing distinct physical and chemical properties, establishing specific ecological niches that are dynamic in nature and vary in different time and space. The composition of microbiomes vary according to different hosts and environments. For example, microbial diversity in the human microbiome is only 10% to that found in the soil microbiome, and the soil microbiome largely consists of bacteria, fungi, archaea, viruses, small protists, and algae (Blum et al., 2019). A gram of soil contains more than 100 million to a billion microorganisms, and less than 1% of these have been characterized (Daniel, 2005; Raynaud & Nunan, 2014). Based on different environments and hosts, microbiomes can be commensal, symbiotic, or parasitic. In this article, we are going to focus on beneficial microbes.
Microbial diversity can be explained as the various range of microbial species, including Bacteria, Archaea, Fungi, Protista, etc. Under the soil ecosystem, bacteria are the most abundant microbial communities, followed by archaea, fungi, etc. (Siles et al., 2018). Microbiomes provide several benefits such as nutrient mobilization and recycling, water holding, organic matter decomposition, high organic carbon and nitrogen content, aeration and fertilizer mobilization, and increased plant productivity and resilience to climate change in agricultural and ecosystem ecology.
Factors Affecting Microbial Activity in Agricultural Systems
Soil microbial diversity varies among different ecosystems and plays a crucial role in regulating nutrient cycling and mobilization, water holding, and organism matter decomposition. The diversity of soil microbes and their functions govern the decomposition of organic matter and release of nutrients, thereby influencing the nutrient availability in the soil. Meanwhile, these activities are highly impacted by soil properties and environmental factors like moisture, soil organic matter, soil texture, pH, precipitation, and aerobic and anaerobic conditions (Girvan et al., 2003; Ghimire et al., 2024).
Soil Characteristics
Various soil textures exhibit different capacities to support microbial communities and their functional characteristics (Sessitch et al., 2001). For example, loam and silt soils typically harbor higher microbial diversity compared with clay and sandy soils. Sandy soils, which are low in organic matter and prone to drought and other environmental stresses, generally host fewer microbes and activity. Moreover, soil pH plays an important role in shaping microbial activity, thereby influencing microbial composition and distributions (Wang et al., 2019). Micro‐ and macroaggregates within the soil also exhibit distinct microbial diversity and distributions. Upton et al. (2019) reported that microaggregates tend to support higher microbial diversity and possess physical properties conducive to enhanced microbial growth and activity, whereas macroaggregates harbor less diverse microbial communities but offer greater stability in their activity. Similarly, soil bulk density also affects microbial diversity; lower bulk density favors more microbial growth, composition, and diversity while higher bulk density tends to suppress microbial activity.
Soil Organic Matter
Soil organic matter is a source of carbon for microorganisms. For example, humus is a big source of carbon and serves as a slow‐release source of carbon and energy. Soil organic matter also contains organic nitrogen, phosphorus, sulfur, and many other elements, and microbes play an important role in recycling these elements through decompositions. The microbes decompose organic compounds to fulfill the microbial nutrient demand. However, the nutrients released from the soil and available to the crop depend on the microbial decomposition and quality of soil organic matter, i.e., the carbon‐to‐nutrient ratio (Bending et al., 2002). Microbial mass makes up to 1–4% of total organic matter; thus, higher organic matter harbors higher microbial compositions and diversity (Bergtold & Sailus, 2020).
Soil Protein
Soil protein represents a crucial reservoir of the organic nitrogen pool within the soil organic matter, which can be metabolized by microbes and released slowly in the usable form for crops across different growth stages (Hurriso et al., 2018). Soil proteins measure the size of the nitrogen pool in the soil (amino acids and other sources) that can be depolymerized into simpler forms and thus indicate the mineralization rate and availability of nitrogen in the soil. Furthermore, soil proteins serve as substrates for microbial activity, thereby fostering microbial activity and influencing microbial composition and diversity. This interplay between soil proteins and microbial dynamics and diversity serves as a reliable predictor for estimating mineralization rates, thereby contributing to nutrient management in crop production (Geisseler et al., 2019). Thus, soil protein content serves as a critical indicator of soil health and microbial dynamics (Naasko et al., 2024).
Climate
Abiotic factors like moisture availability, precipitation, and temperature exert strong influences on microbial diversity and activity in soil ecosystems. Borowik and Wyszkowska (2016) observed the varied impact of moisture levels on soil microbial communities, abundance, and activities. Climate change, with its multifaceted impacts on agriculture and ecosystems, introduces profound impacts on soil microbiomes. Elevated carbon dioxide levels, for instance, have been associated with reduced microbial diversity (Yang et al., 2019), alongside increased mycorrhizal activity and abundance (Dhillion et al., 1995). Changes in precipitation patterns further disrupt soil microbial growth, altering the rate of the soil organic matter decomposition and nutrient cycling. For instance, drought stress exacerbates these effects by modifying soil moisture levels and soil chemistry, impacting soil microbiomes (Naylor & Coleman Derr, 2018). While elevated temperatures initially enhance microbial activity and biomass, prolonged exposure leads to shifts in microbial distribution, diversity, and functional composition (Rasmussen et al., 2020; Zogg et al., 1997). These environmental conditions drive shifts in microbial diversity across diverse agro‐ecological systems.
Crop Species and Diversity
Crop species play a pivotal role shaping microbial communities, fostering microbial–plant associations and influencing key functions within agroecosystems. Crop species like grasses, pulses, and other species hold different levels of potential to harbor different microbial communities. Mixed cropping with cereals and legumes increases microbial diversity and composition, facilitating plant‐microbe associations (Stefan et al., 2021). Understanding the role of crop species in shaping the microbiome will help in managing farm‐level decisions and adopting better practices in different soil and environments in agricultural systems. For example, certain forage grasses have been found to enhance bacterial and fungal diversity, simultaneously increasing nitrifying bacteria like archaea and decreasing denitrifying bacteria (Momesso et al., 2022). Conversely, the cultivation of pulses has shown to augment fungal communities, particularly ascomycetes and basidiomycetes (Yang et al., 2021), whereas cereals like rye can stimulate bacterial communities such as diazotrophs and denitrifying bacteria (Lewin et al., 2024). Thus, diversification of crop species is a promising strategy for fostering microbial diversity and abundance.
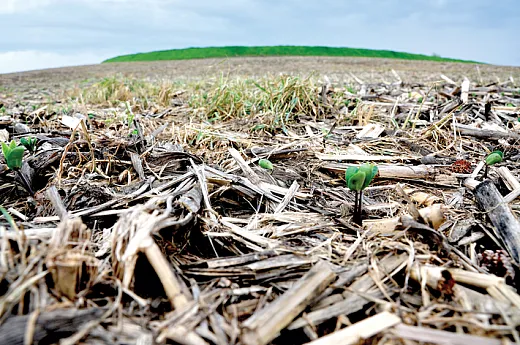
Cultural Practices
Cultural practices such as crop rotation, mulching, cover cropping, intercropping and conservation tillage serve to diversify the soil microbiome, thereby enhancing microbial activity and mineralization processes (Xie et al., 2022). Town et al. (2023) reported that crop rotation schemes incorporating canola, wheat, barley, and pea exert a significant influence on rhizosphere microbiome composition. Similarly, Sun et al. (2023) observed that crop rotation involving pulses led to increased soil carbon and nutrient pools, ultimately enhancing crop productivity. Mulching is also one of the important cultural practices for improving soil moisture retention and reducing moisture evaporation, with profound impacts on soil microbiomes. Wang et al. (2020) reported that mulching enhances soil fungal activity and nitrogen availability. Furthermore, mulching influences temperature, regulates soil aeration, and increases moisture availability, thereby impacting soil chemistry, organic matter decomposition, and nutrient recycling (Shan et al., 2022; Tian et al., 2022). Innovative land management strategies, such as topsoil replacement in eroded soils, have been shown to bolster soil microbial activities, enhance soil health, and increase crop yield (Schneider et al., 2023). Identifying the suitable cultural practices based on crop, environmental, and soil conditions will help to bolster microbial activity and their compositions.
External Inputs
External inputs such as pesticides, fertilizers, and various inoculants play significant roles in modern agriculture practices, yet their impacts on soil microbial activity and abundance vary. Numerous studies have shown that pesticide application, whether foliar or soil based, reduces bacterial diversity and their abundance (Onwona‐Kwakye et al., 2020; Jeyaseelan et al., 2023). Similarly, the effects of fertilization are multifaceted; while long‐term studies spanning 55 years have shown no significant impact on soil microbial biomass and community composition, short‐term effects yield either positive or negative effects on microbial biomass and abundance, which are also dependant on fertilizer dosage (Williams et al., 2013; O’donnell et al., 2001). Organic inputs like biochars and manure applications contribute to enrichment of soil organic matter, consequently bolstering the diversity and abundance of microbial communities, thus helping nutrient recycling and improving crop production (Nielsen et al., 2014). Additionally, the introduction of native microorganisms into degraded soils has been found to enhance soil function and microbial compositions (Dadzie et al., 2024) while microbial inoculants have demonstrated a positive effect on plant and soil health as well as microbial diversity and abundance (Alori et al., 2017). Thus, suitable external inputs can be amended in soil to improve the soil microbial activity and abundance.
Overall, adopting good management practices is vital for establishing the strong foundation for a sustainable future of microbial ecology and nurturing its multifaceted interactions with different components of the soil and environment. Focusing on microbial diversity presents both opportunities and responsibilities for improving and adopting various agricultural practices. Given the pressing issues of global warming, greenhouse gas emissions, and heavy reliance on chemical fertilizers and pesticides in modern agriculture, urgent actions are imperative to mitigate their adverse effects on agriculture and public health. Furthermore, the diverse microbial community plays a crucial role in promoting the health of humans, animals, plants, and soil, aligning with objectives of initiatives like the One Health Initiative (Banerjee & Van Der Heijden, 2023). Thus, prioritizing microbial diversity fosters sustainability, resilience, and innovation across diverse fields, addressing critical environmental, agricultural, health, and industrial challenges and laying the foundations for holistic solutions towards a sustainable future.
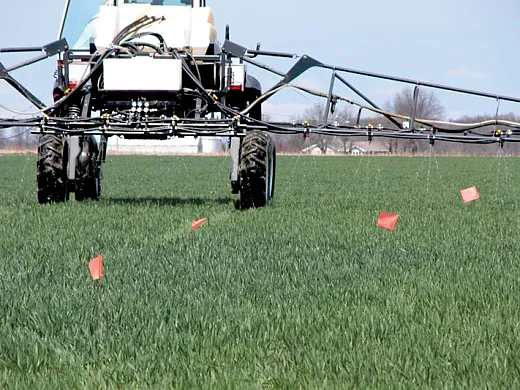
References
Alori, E. T., Dare, M. O., & Babalola, O. O. (2017). Microbial inoculants for soil quality and plant health. Sustainable agriculture reviews, 22, 281–307.
Banerjee, S., & Van Der Heijden, M. G. (2023). Soil microbiomes and one health. Nature Reviews Microbiology, 21(1), 6–20.
Bending, G. D., Turner, M. K., & Jones, J. E. (2002). Interactions between crop residue and soil organic matter quality and the functional diversity of soil microbial communities. Soil Biology and Biochemistry, 34(8), 1073–1082.
Bergtold, J., & Sailus, M. (2020). Conservation tillage systems in the Southeast: production, profitability and stewardship. Sustainable Agriculture Research and Education (SARE) Program.
Blum, W. E., Zechmeister‐Boltenstern, S., & Keiblinger, K. M. (2019). Does soil contribute to the human gut microbiome? Microorganisms, 7(9), 287.
Borowik, A., & Wyszkowska, J. (2016). Soil moisture as a factor affecting the microbiological and biochemical activity of soil.
Dadzie, F. A., Moles, A. T., Erickson, T. E., Machado de Lima, N., & Muñoz‐Rojas, M. (2024). Inoculating native microorganisms improved soil function and altered the microbial composition of a degraded soil. Restoration Ecology, 32(2), e14025.
Daniel, R. (2005). The metagenomics of soil. Nature Reviews Microbiology, 3(6), 470–478.
Dhillion, S. S., Roy, J., & Abrams, M. (1995). Assessing the impact of elevated CO2 on soil microbial activity in a Mediterranean model ecosystem. Plant and Soil, 187, 333–342.
Geisseler, D., Miller, K., Leinfelder‐Miles, M., & Wilson, R. (2019). Use of soil protein pools as indicators of soil nitrogen mineralization potential. Soil Science Society of America Journal, 83(4), 1236–1243.
Ghimire, O. P., Nepal, J., Poudel, P., & Powar, S. (2024). Unlocking the secrets of soil: Exploring the microbiome and its applications—Part 1. CSA News, 69(4), 12–18.
Girvan, M. S., Bullimore, J., Pretty, J. N., Osborn, A. M., & Ball, A. S. (2003). Soil type is the primary determinant of the composition of the total and active bacterial communities in arable soils. Applied and environmental microbiology, 69(3), 1800–1809.
Hurisso, T. T., Moebius‐Clune, D. J., Culman, S. W., Moebius‐Clune, B. N., Thies, J. E., & van Es, H. M. (2018). Soil protein as a rapid soil health indicator of potentially available organic nitrogen. Agricultural & Environmental Letters, 3(1), 180006.
Jeyaseelan, A., Murugesan, K., Thayanithi, S., & Palanisamy, S. B. (2023). A review of the impact of herbicides and insecticides on the microbial communities. Environmental Research, 245, 118020.
Lewin, S., Wende, S., Wehrhan, M., Verch, G., Ganugi, P., Sommer, M., & Kolb, S. (2024). Cereals rhizosphere microbiome undergoes host selection of nitrogen cycle guilds correlated to crop productivity. Science of The Total Environment, 911, 168794.
Momesso, L., Crusciol, C. A., Leite, M. F., Bossolani, J. W., & Kuramae, E. E. (2022). Forage grasses steer soil nitrogen processes, microbial populations, and microbiome composition in a long‐term tropical agriculture system. Agriculture, Ecosystems & Environment, 323, 107688.
Naasko, K., Martin, T., Mammana, C., Murray, J., Mann, M., & Sprunger, C. (2024). Soil protein: A key indicator of soil health and nitrogen management. Soil Science Society of America Journal, 88(1), 89–108.
Naylor, D., & Coleman‐Derr, D. (2018). Drought stress and root‐associated bacterial communities. Frontiers in Plant Science, 8, 303756.
Nielsen, S., Minchin, T., Kimber, S., van Zwieten, L., Gilbert, J., Munroe, P., … & Thomas, T. (2014). Comparative analysis of the microbial communities in agricultural soil amended with enhanced biochars or traditional fertilisers. Agriculture, Ecosystems & Environment, 191, 73–82.
O’donnell, A. G., Seasman, M., Macrae, A., Waite, I., & Davies, J. T. (2001). Plants and fertilisers as drivers of change in microbial community structure and function in soils. Plant and Soil, 232, 135–145.
Onwona‐Kwakye, M., Plants‐Paris, K., Keita, K., Lee, J., Brink, P. J. V. D., Hogarh, J. N., & Darkoh, C. (2020). Pesticides decrease bacterial diversity and abundance of irrigated rice fields. Microorganisms, 8(3), 318.
Rasmussen, P. U., Bennett, A. E., & Tack, A. J. (2020). The impact of elevated temperature and drought on the ecology and evolution of plant–soil microbe interactions. Journal of Ecology, 108(1), 337–352.
Raynaud, X., & Nunan, N. (2014). Spatial ecology of bacteria at the microscale in soil. PloS one, 9(1), e87217. Schneider, S. K., Sutradhar, A. K., Duke, S. E., Lehman, R. M.,
Schumacher, T. E., & Lobb, D. A. (2023). Key soil properties and their relationships with crop yields as affected by soil–landscape rehabilitation. Agronomy Journal, 115(5), 2404–2423.
Sessitsch, A., Weilharter, A., Gerzabek, M. H., Kirchmann, H., & Kandeler, E. (2001). Microbial population structures in soil particle size fractions of a long‐term fertilizer field experiment. Applied and Environmental Microbiology, 67(9), 4215–4224.
Shan, X., Zhang, W., Dai, Z., Li, J., Mao, W., Yu, F., … & Zeng, X. (2022). Comparative analysis of the effects of plastic mulch films on soil nutrient, yields and soil microbiome in three vegetable fields. Agronomy, 12(2), 506.
Siles, J. A., Öhlinger, B., Cajthaml, T., Kistler, E., & Margesin, R. (2018). Characterization of soil bacterial, archaeal and fungal communities inhabiting archaeological human‐impacted layers at Monte Iato settlement (Sicily, Italy). Scientific Reports, 8(1), 1903.
Stefan, L., Hartmann, M., Engbersen, N., Six, J., & Schöb, C. (2021). Positive effects of crop diversity on productivity driven by changes in soil microbial composition. Frontiers in Microbiology, 12, 660749.
Sun, L., Wang, S., Narsing Rao, M. P., Shi, Y., Lian, Z. H., Jin, P. J., … & Wei, D. (2023). The shift of soil microbial community induced by cropping sequence affect soil properties and crop yield. Frontiers in Microbiology, 14, 1095688.
Tian, L., Yu, S., Zhang, L., Dong, K., & Feng, B. (2022). Mulching practices manipulate the microbial community diversity and network of root‑associated compartments in the Loess Plateau. Soil and Tillage Research, 223, 105476.
Town, J. R., Dumonceaux, T., Tidemann, B., & Helgason, B. L. (2023). Crop rotation significantly influences the composition of soil, rhizosphere, and root microbiota in canola (Brassica napus L.). Environmental Microbiome, 18(1), 40.
Upton, R. N., Bach, E. M., & Hofmockel, K. S. (2019). Spatio‐temporal microbial community dynamics within soil aggregates. Soil Biology and Biochemistry, 132, 5–68.
Wang, C. Y., Zhou, X., Guo, D., Zhao, J. H., Yan, L., Feng, G. Z., … & Zhao, L. P. (2019). Soil pH is the primary factor driving the distribution and function of microorganisms in farmland soils in northeastern China. Annals of Microbiology, 69(13), 1461–1473.
Wang, Y., Liu, L., Luo, Y., Awasthi, M. K., Yang, J., Duan, Y., … & Zhao, Z. (2020). Mulching practices alter the bacterial‐fungal community and network in favor of soil quality in a semiarid orchard system. Science of the Total Environment, 725, 138527.
Williams, A., Börjesson, G., & Hedlund, K. (2013). The effects of 55 years of different inorganic fertiliser regimes on soil properties and microbial community composition. Soil Biology and Biochemistry, 67, 41–46.
Xie, Y., Ouyang, Y., Han, S., Se, J., Tang, S., Yang, Y., … & Wu, L. (2022). Crop rotation stage has a greater effect than fertilisation on soil microbiome assembly and enzymatic stoichiometry. Science of the Total Environment, 815, 152956.
Yang, S., Zheng, Q., Yuan, M., Shi, Z., Chiariello, N. R., Docherty, K. M., … & Yang, Y. (2019). Long‐term elevated CO2 shifts composition of soil microbial communities in a Californian annual grassland, reducing growth and N utilization potentials. Science of the Total Environment, 652, 1474–1481.
Yang, T., Evans, B., & Bainard, L. D. (2021). Pulse frequency in crop rotations alters soil microbial community networks and the relative abundance of fungal plant pathogens. Frontiers in Microbiology, 12, 667394.
Zogg, G. P., Zak, D. R., Ringelberg, D. B., White, D. C., MacDonald, N. W., & Pregitzer, K. S. (1997). Compositional and functional shifts in microbial communities due to soil warming. Soil Science Society of America Journal, 61(2), 475–481.
Text © . The authors. CC BY-NC-ND 4.0. Except where otherwise noted, images are subject to copyright. Any reuse without express permission from the copyright owner is prohibited.