Heat Stress in Canola: Paving a Path Forward for Varieties With Improved Tolerance
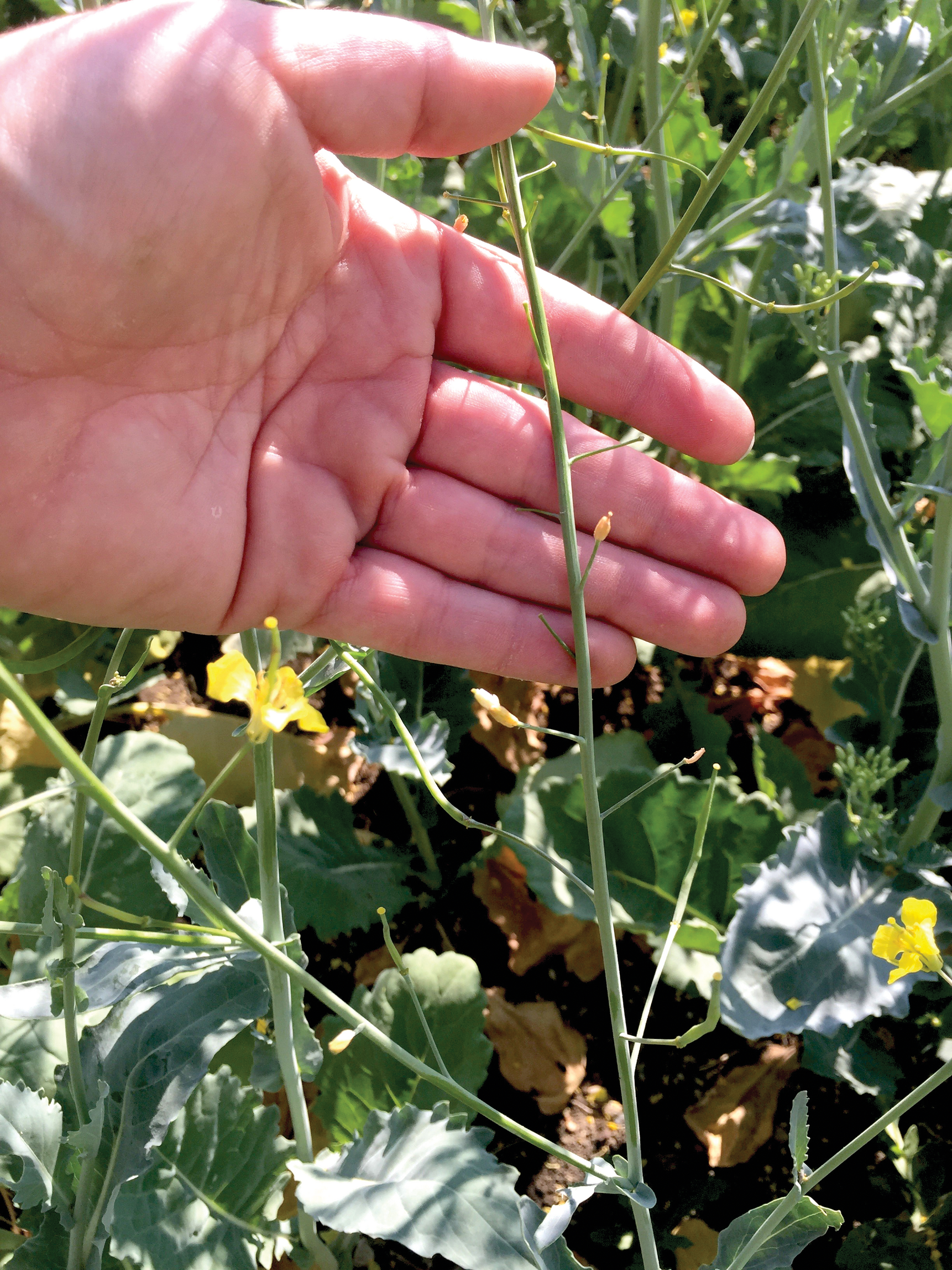
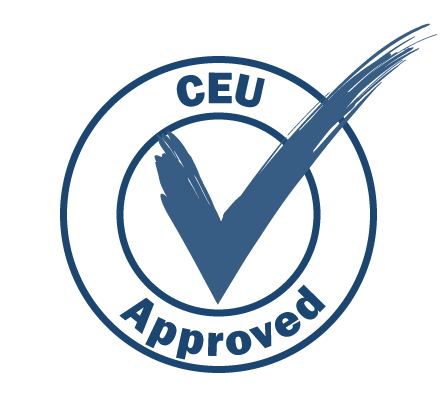
Canola grown in years or locations with increased temperatures shows a significant decline in seed yield. Research has shown a ∼7 bu/ac yield loss with a 5.4°F increase in growing season temperatures. Given the importance of canola in western Canada and the fact that canola is a cool-season crop, continued applied and basic research into heat tolerance is of critical importance. Earn 0.5 CEUs in Crop Management by reading this article and taking the quiz at https://web.sciencesocieties.org/Learning-Center/Courses.
A study conducted for the Canola Council of Canada in December 2020 reported that the canola industry in Canada contributed an economic impact of $29.9 billion. Given the importance of canola in western Canada and the fact that canola is a cool-season crop, continued applied and basic research into heat tolerance is of critical importance. Canola grown in years or locations with increased temperatures shows a significant decline in seed yield. Nuttal et al. (1992) observed a ~7 bu/ac yield loss with a 5.4°F increase in growing season temperatures.
Heat stress can be defined in multiple ways, such as a sustained increase in average temperatures, transient heat waves, or sporadic days of high temperature. Research has shown that all of these stresses can negatively impact canola yield. Interestingly, the western Canadian spring canola crop is the only region in the world where canola flowers during the hottest temperatures of the year. In other important canola or winter rapeseed-growing regions, canola flowers in the spring and thus escapes much of the summer heat.
Given canola’s sensitivity to heat stress and its flowering time in the heat of the summer, questions need to be answered regarding heat tolerance in canola. First, we wanted to determine if there was genetic variation within spring canola germplasm for heat stress. Secondly, with the transition from open-pollinated cultivars to hybrids over the past two decades, we investigated the impact of heterosis on improving heat stress tolerance. Finally, different breeding methodologies were examined to determine a path forward for developing heat-tolerant canola.
Corteva Agriscience, along with the University of Manitoba, attempted to answer these questions in a collaboration from 2014–2019. The collaboration leveraged the University of Manitoba’s research space and expertise, along with Corteva’s global canola germplasm, to explore heat tolerance in canola. Previous canola heat tolerance research conducted at the University of Manitoba by Dr. Malcolm Morrison enabled the project to use his previously published methods (Morrison, 1993).
These previously described indoor screening methods provided an excellent framework for further investigation to determine if there was variation to heat tolerance existing in spring canola germplasm. Previous literature points to the early reproductive stage as being the most sensitive to heat stress.
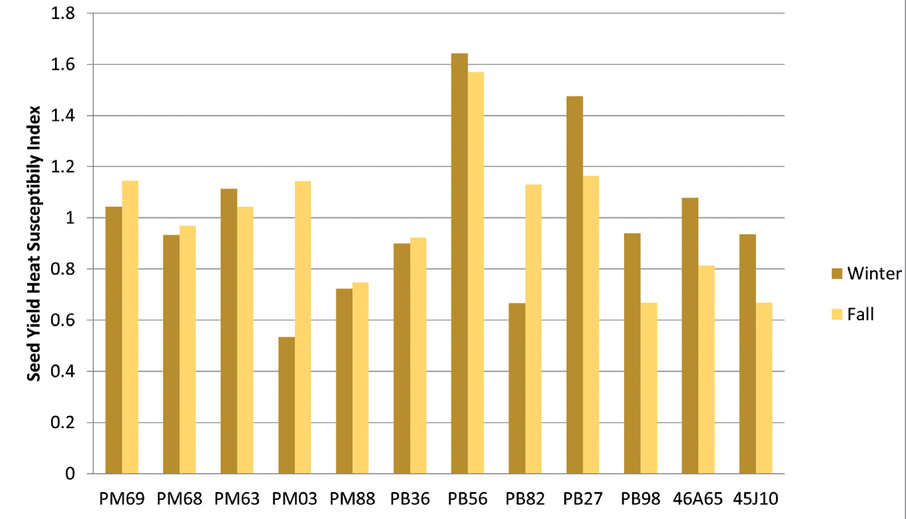
Experiment Details
Genotypes were grown in greenhouse conditions with the high temperature set to 73°F. At the rosette stage, plants were moved to either a heat stress growth chamber or a control growth chamber for 14 days. Once the treatment was complete, the plants were then moved back to the greenhouse for the remainder of the life cycle.
A heat susceptibility index (HSI) was calculated, where HSI = (1 - Yh / Yc) / (1 - Xh / Xc), with Yh being the yield of the genotype exposed to the heat stress, Yc the yield of the genotype grown in control temperatures, Xh the mean of the entries exposed to the heat stress, and Xc the mean of the entries exposed to the control temperatures. The HSI was utilized to differentiate canola inbred response to indoor heat stress. The lower the score, the better the heat tolerance. Genotypes with a score of greater than 1 were heat susceptible.
Figure 1 illustrates the HSI differences within the genotypes tested. There were genotypes within the experiment that demonstrated significant heat tolerance compared with 45J10 (Brassica juncea), a species recognized for its heat tolerance. It should be noted that this experiment was not water-limited, and along with being recognized for its heat tolerance, B. juncea is known for being more drought-tolerant than canola. This set of experiments was designed to elucidate differences in heat tolerance under conditions where soil moisture was not limited.
Differences in Developmental and Yield Component Characteristics in Heat-Stressed Canola
Several different traits were measured in an attempt to determine the factors that led to the reduction in yield (Table 1). Heat-stressed canola produces more flowers, yet sets fewer seed-bearing pods, resulting in a significantly lower flower-to-pod ratio. Fewer flowers resulting in seed-bearing pods, along with greater final biomass, reduced the overall harvest index in the heat-stressed treatments.
Table 1. Developmental and yield component characteristics per plant for canola grown under control and heat treatments in the greenhouse and growth chamber at the University of Manitoba, with significance across treatments tested using a paired t-test.
| Winter | Fall | ||||
---|---|---|---|---|---|---|
Trait | Control
| Heat | Percent | Control | Heat | Percent |
Days to Growth Stage 53 | 36 | 39* | 8.3 | 39 | 43* | 10.3 |
Days to first flower | 39 | 42* | 7.7 | 44 | 47* | 6.8 |
Days to last flower 62 | 62 | 69* | 11.3 | 75 | 80* | 6.7 |
Flower duration | 23 | 27* | 17.4 | 32 | 33 | 3.1 |
Total flower number | 180 | 223* | 23.9 | 161 | 186* | 15.5 |
Total pod number | 104 | 70* | −32.7 | 85 | 63* | −25.9 |
Pod-to-flower ratio | 0.59 | 0.34* | −42.4 | 0.53 | 0.37* | −30.2 |
Shoot biomass (g) | 15.8 | 18.2* | 15.2 | 12.6 | 10.9* | −13.5 |
Pollen number | 98 | 43* | −56.1 | 93 | 52* | −44.1 |
Seed number | 1280 | 412* | −67.8 | 969 | 453* | −53.3 |
Seed per pod | 13 | 6* | −53.8 | 14 | 7* | −50.0 |
Thousand-kernel weight (g) | 3.04 | 4.4* | 44.7 | 2.39 | 2.96 | 23.8 |
Yield (g) | 3.77 | 1.68* | −55.4 | 2.2 | 1.30* | −40.9 |
Harvest index | 0.25 | 0.11* | −56.0 | 0.18 | 0.12* | −33.3 |
*p < 0.05.
Pollen number was assessed in the control and heat-stressed genotypes, and unsurprisingly, the heat treatment had significantly fewer pollen grains. This is likely one of the main contributing factors to the lower flower-to-pod ratio. This explains some of the reduction in seed set. Other researchers have demonstrated that heat stress can cause pollen grains to produce less vigorous pollen tubes or become nonviable. The combination of fewer total pollen grains, fewer viable pollen grains, and less vigorous pollen tubes all likely play a role in fewer seeds being set under heat stress.
Can Existing Genetic Variation Be Used to Create Hybrids With Differing Heat Stress Responses?
Given that heat tolerance variation within spring canola germplasm was present, the next step was to assess whether this variation within inbreds could create hybrids with differing responses to heat stress. The inbred and hybrid genotypes were tested in field plots using planting date as a proxy for the heat treatment. The expectation was that the later-planted treatment would be exposed to higher temperatures during flowering. Two locations across two years for a total of four sites-years of data were generated, and a principal component analysis was used to determine which weather variables had the most significant impact on yield.
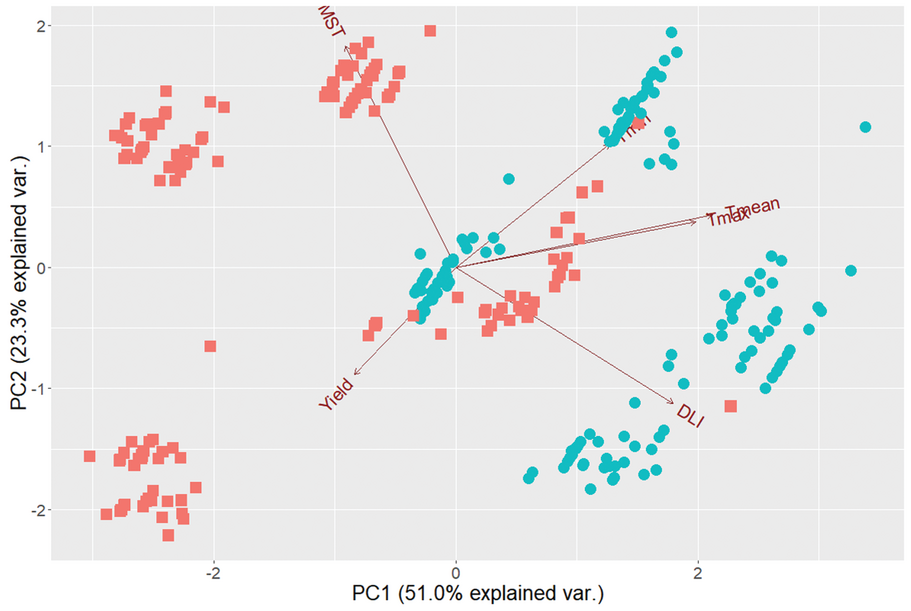
Figure 2 illustrates that higher maximum and minimum temperatures had a direct negative impact on yield while soil moisture and light intensity had much less of an impact on overall yield. The late- planted treatment (heat proxy) had a 23% lower seed yield on average (Table 2). Interestingly, flower and pod number were not significantly different between the planting dates. Seed size was 7.5% larger in the earlier planting date, which explains a portion of the higher yield with the remainder likely being attributed to the number of seeds within each pod. Earlier planting also raised oil content and lowered protein, glucosinolates, and saturates. The average inbred yield was reduced by 25% in the heat treatment while the average hybrid yield was reduced by 20%. This 5% difference aligns with the often-reported stress buffering capacity of hybrids in multiple crops.
| Early | Late | Difference |
---|---|---|---|
Days to flower beginning | 47 | 41 | 61* |
Days to flower ending | 74 | 63 | 111* |
Flower duration (days) | 27 | 22 | 51* |
Flower number | 141 | 138 | 3ns |
Pod number | 101 93 | 93 | 8ns |
Days to maturity | 97 87 | 87 | 101* |
Seed yield (lb/ac) | 1742 | 1346 | 3961* |
Thousand-kernel weight (g) | 3.14 | 92 | 0.221* |
Percent oil | 46.36 | 44.74 | 1.621* |
Percent protein | 25.64 | 26.93 | −1.291* |
Glucosinolates (μmol/g) | 14.64 | 16.11 | −1.471* |
Saturates (% of total fatty acids) | 6.57 | 6.75 | −0.181* |
In attempting to improve stress tolerance in commercial hybrids, it is necessary that there is no reduction in yield when the stress is not present. Given heat stress can be sustained, transient, or sporadic, heat-stress-tolerant hybrids need to deliver high yields under optimum conditions. In reviewing the hybrid performance across these four locations, it was noted that some hybrids had improved performance in the late planting as compared with others. These hybrids were near the top in performance; and as seen in Figure 3, there were several crossovers where hybrids improved in ranking under the late planting. This increase in stability of performance can be a benefit in areas that experience heat stress more frequently.
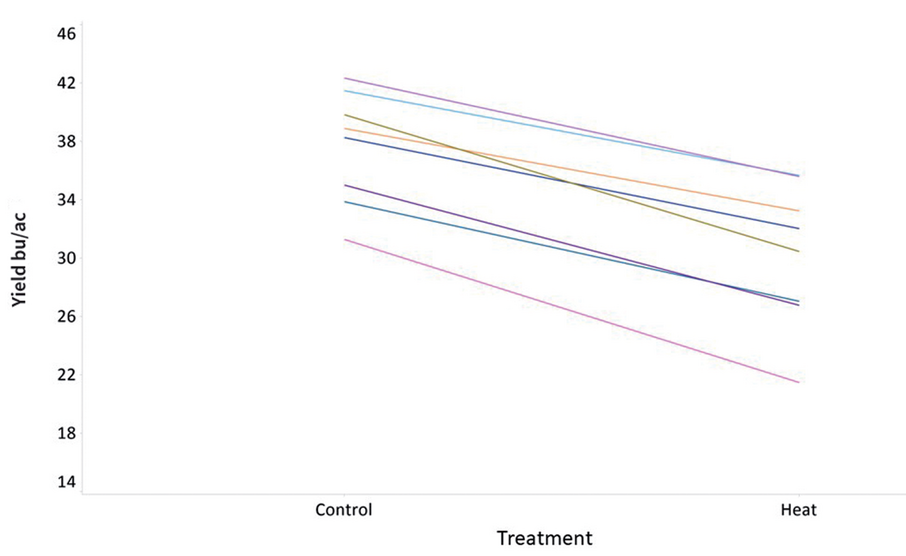
The ability to make consistent genetic gains for heat tolerance requires repetitive ability to differentiate germplasm and select a breeding method that best aligns with the trait of interest. Several studies have demonstrated that heat stress response is highly quantitative, involving many genes and regulatory pathways. With this understanding, utilization of whole-genome predictions may be the breeding method that will enable breeders to improve heat tolerance in the coming years. While whole-genome predictions have been shown to be extremely useful in making gain for highly quantitative traits such as yield, robust phenotypic data is required to enable selection gains. Due to this, phenotyping for heat tolerance should be an increased focus for those looking to make heat tolerance improvements. There are numerous different heat stress phenotyping methods in the literature, and given the various definitions of heat stress, it is unlikely that a single method will enable screening for all heat stress definitions. The low hanging fruit will be to make progress on the most susceptible stages where there is the most variation within the germplasm.
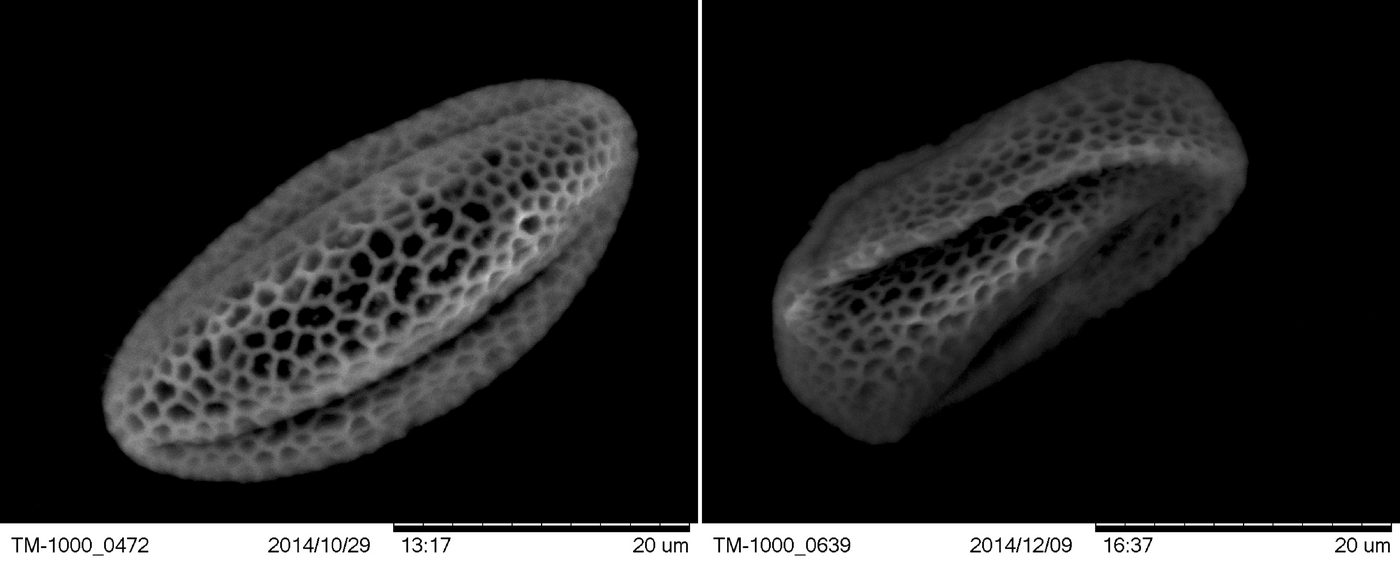
There is ample room for further understanding of the mechanism of heat tolerance and other basic research questions within this area. Further investigation was required into the impact of heat stress on pollen grains as pollen develops. The research project attempted to discover changes in the pollen grain morphology using scanning electron microscopy. No differences could be attributed to genetics although deformed pollen morphology was evident within the heat stress treatments and further research into heat stress pollen is required (Figure 4).
Along with this basic research requirement, further understanding of scalable and repeatable heat tolerance selection will be required. Repeatable selection needs to be made in each generation to enable consistent genetic gain across parent cycles and a well-characterised field response can be predicted in the target population and environment. This could be conducted through sufficient field testing in well-characterised environments where heat stress is the limiting factor.
This aligns well with private companies that specialize in running testing plots on a large scale and across a broad geography. Close relationships between universities and private companies enable a multi-faceted approach to solving complex problems such as heat stress tolerance. In the end, all parties benefit from one another’s expertise and the ability to advance science and knowledge improves.
How Can Management Play a Role?
While genetic enhancements and further basic understanding into the physiology surrounding canola heat tolerance is required, growers can adjust their practices to enable their canola crop to escape the heat and or hedge the impact of transient or sporadic heat events through adjusting planting date. Given the unpredictable nature of heat stress events, growers can spread their planting dates across multiple weeks to ensure that not all their crop will be negatively impacted if a heat wave hits the early reproductive stages. In doing so, growers can also minimize other stresses (abiotic or biotic) as the literature clearly points to multiple stresses compounding and leading to significantly greater yield loss.
References
Koscielny, C. B. (2018). Analysis of thermotolerance in Brassica napus L. Morrison, M.J. (1993). Heat stress during reproduction in summer rape. Canadian Journal of Botany, 71, 303–308. https://doi.org/10.1139/b93-031.
Nuttall, W.F., Moulin, A.P., & Townley-Smith, L.J. (1992). Yield response of canola to nitrogen, phosphorus, precipitation, and temperature. Agronromy Journal, 84, 765–768. https://doi.org/10.2134/agronj1992.00021962008400050001x.
Self-Study CEU Quiz
Earn 1 CEU in Nutrient Management by taking the quiz for the article at https://web.sciencesocieties.org/Learning-Center/Courses. For your convenience, the quiz is printed below. The CEU can be purchased individually, or you can access as part of your Online Classroom Subscription.
- In which region of the world does spring canola flower during the hottest temperatures of the year?
- China.
- The U.S. state of Idaho.
- Western Europe.
- Western Canada.
- Heat stress is narrowly defined as a sudden increase in high temperatures, as opposed to a sustained increase in average temperatures.
- True.
- False.
- How did the flower-to-pod ratio differ between canola grown under control and heat treatments?
- The heat-stressed canola produced more flowers and more pods than the control.
- The heat-stressed canola produced fewer flowers and fewer pods than the control.
- The heat-stressed canola produced fewer flowers and more pods than the control.
- The heat-stressed canola produced more flowers and fewer pods than the control.
- The later-planted treatment, which was exposed to higher temperatures during flowering, had a ____ seed yield than the early-planted treatment.
- 23% larger.
- 23% lower.
- 7.5% larger.
- 7.5% lower.
- The researchers found deformed morphology in pollen grains from the heat stress treatment.
- True.
- False.
Text © . The authors. CC BY-NC-ND 4.0. Except where otherwise noted, images are subject to copyright. Any reuse without express permission from the copyright owner is prohibited.