Regenerative Practices Show Promise in Dryland Cotton Systems
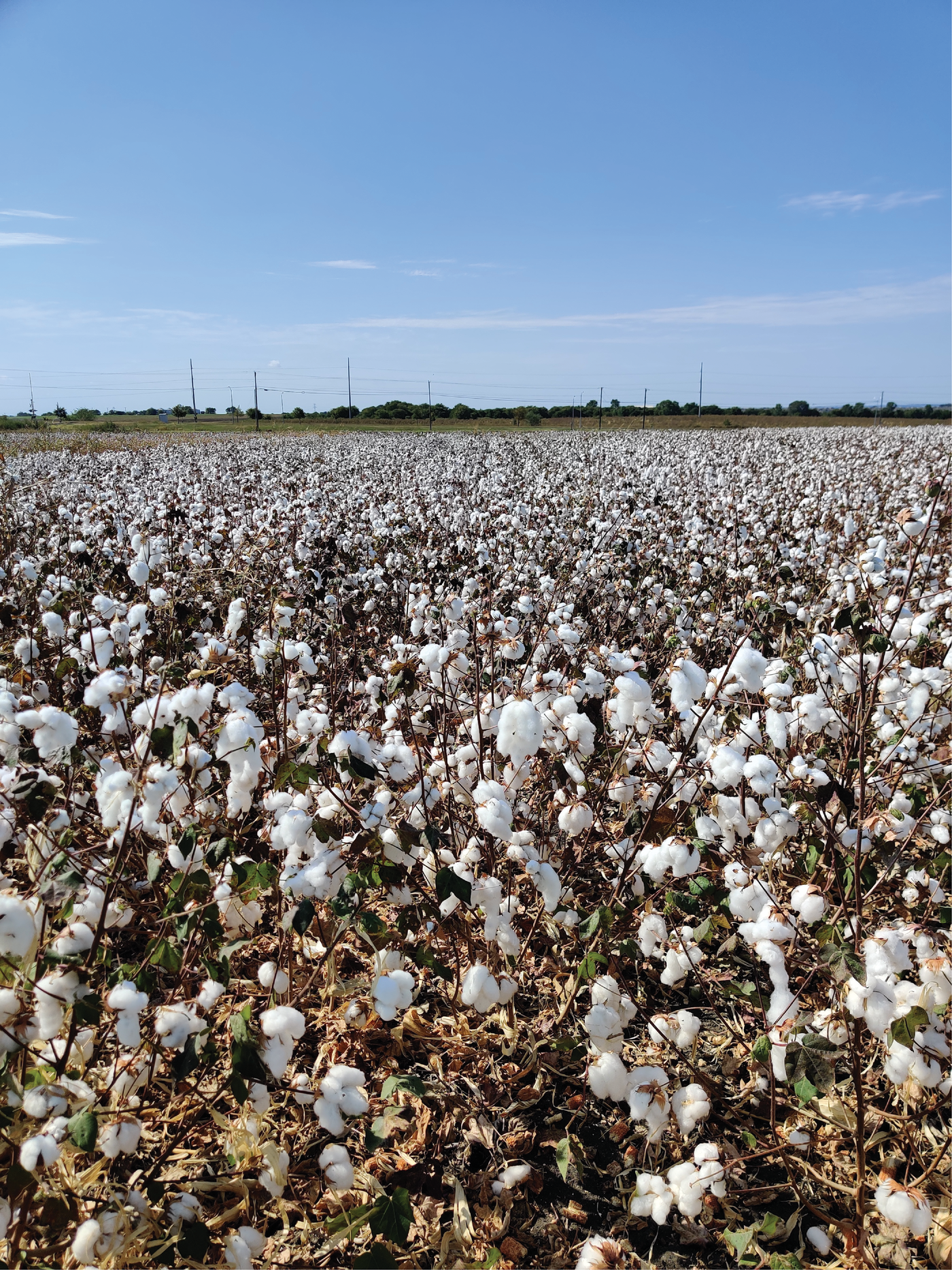
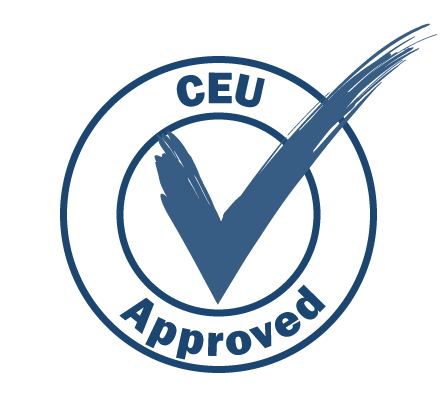
Regenerative practices, such as no-till and cover crops, are advocated for their positive impacts on soil health and the environment. Nevertheless, there are challenges associated with implementing these practices. The objective of this simulation study was to assess the potential for soil carbon sequestration, soil water storage, and cotton yield response when implementing regenerative practices in the water-limited environment of the Texas Rolling Plains region. Earn 1 CEU in Sustainability by reading this article and taking the quiz.
Regenerative practices, such as no-till and cover crops, are advocated for their positive impacts on soil health and the environment. Nevertheless, there are challenges associated with implementing these practices. The objective of this simulation study was to assess the potential for soil carbon sequestration, soil water storage, and cotton yield response when implementing regenerative practices in the water-limited environment of the Texas Rolling Plains region. Earn 1 CEU in Sustainability by reading this article and taking the quiz.
The Texas Rolling Plains (TRP) region is located at the eastern edge of the Southern Great Plains (SGP), where water stands out as the most limiting factor in crop production. Cotton holds significant economic importance in the TRP, contributing 13% of cotton production in the state (DeLaune et al., 2012). While most cotton production in this region is rainfed, with an average annual precipitation of 27 inches, only 16% of planted cotton is irrigated. Producers in this region are interested in regenerative practices to improve soil health and water-use efficiency, but the adoption of regenerative practices remains low compared with other cotton-producing regions. For example, only 30% of cotton in the SGP region is under conservation tillage, which is in stark contrast to the humid southeastern U.S., where about 70% is planted under conservation tillage. Typically, cotton production involves an average of 7 to 12 tillage operations throughout the year with minimal aboveground residue inputs after harvest (Figure 1). Surface residue management is critical in the TRP region due to semi-arid climatic conditions, including highly variable precipitation, intermittent drought stress, temperature extremes, and strong winds.
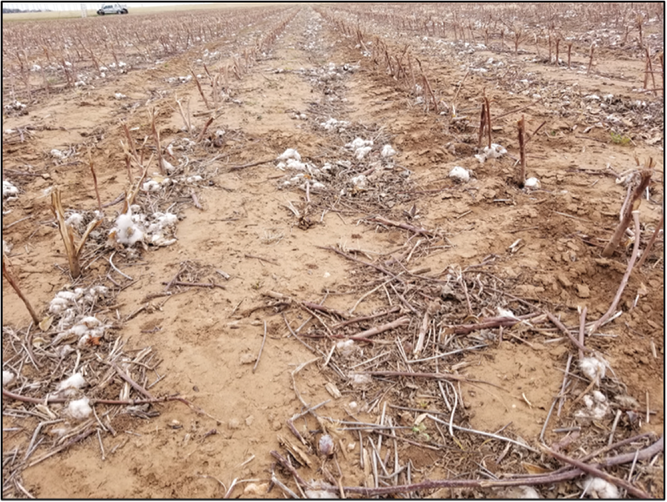
Cover cropping could potentially benefit soil health by adding above- and belowground residue during the winter fallow period. The residue cover, coupled with the no-till practice, could protect young plants from erosion-related damage, increase infiltration, and reduce evaporation losses. This, in turn, may facilitate greater precipitation storage. However, concerns arise about the effects of cover crops on depleting soil water content, increasing competition for nutrients, and potentially impacting the subsequent cotton crop.
Assessing the full potential of regenerative practices on soil and crop productivity requires many years of crop growing season data under varied weather conditions. Such long-term field experiments are limited in the TRP and SGP. Simulation studies, like the one conducted by researchers at the Texas A&M AgriLife Research and Extension Center at Vernon, are useful to complement the results from field experiments where observed data are limited. In this study (Singh et al., 2023), the researchers utilized a biogeochemical model, DeNitrification-DeComposition (DNDC), in conjunction with the data from a nine-year field experiment to understand the long-term effects of regenerative practices on soil carbon sequestration, soil water storage, and cotton yield.
Methods Description
The cover crop field experiment was initiated in fall 2011 near Chillicothe, TX, on Granfield fine sandy loam soil. This experiment included five winter cover crop treatments and two controls under a continuous dryland cotton cropping system. The treatments included no-till with five cover crops: Austrian winter pea, hairy vetch, crimson clover, winter wheat, and a multispecies mixture of grasses and legumes. The control treatments (without cover crop) comprised conventional tillage (disking in fall and spring with additional in-season cultivation using sweeps to a depth of 4–6 inches) and no tillage. Cotton was planted as the cash crop each year, harvested for seed cotton yield (seeds plus lint), and the remaining stalks were shredded. Cover crops were planted with a no-till drill after cotton harvest and chemically terminated in mid- to late April. Notably, no fertilizer or irrigation was applied to either cotton or cover crops during the study. More detailed information about the field experiment can be found in DeLaune & Mubvumba (2020) and DeLaune et al. (2020).
Field data collected from 2011 to 2020 included soil moisture to a depth of 2.6 ft, stocks of soil organic carbon and total nitrogen to a depth of 2 ft, and cover crop biomass, nitrogen uptake, and cotton yield. This extensive field data set was divided into two parts, with about half of the data utilized for model training (i.e., calibration) and the remaining data for model testing (i.e., validation). Following a rigorous calibration and validation process, the DNDC model was employed for conducting long-term simulations, enabling a comprehensive understanding of the impacts of no-till and cover crops on soil organic carbon and cotton yield. In addition, simulations were also conducted to study the effect of different termination dates of cover crops on cotton yield, cover crop biomass, and soil water storage.
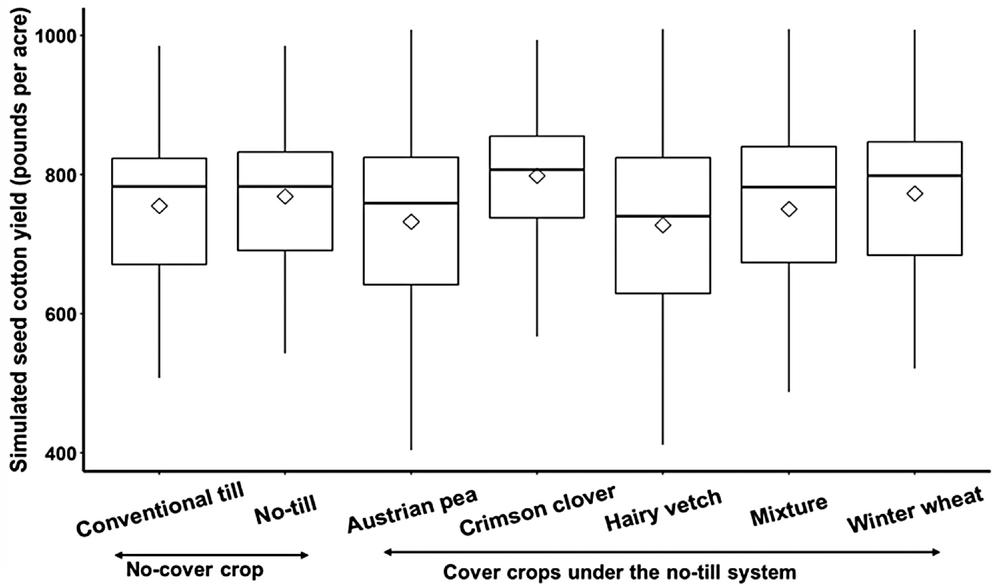
Regenerative Practices and Cotton Productivity
An accurate estimation of plant biomass and carbon-to-nitrogen (CN) ratio is crucial in the simulations as it provides essential input for soil organic matter storage, thereby integrating the plant and soil components into a cohesive biogeochemical system. The DNDC model successfully simulated cover crop biomass and N uptake with error margins within 3% and 13%, respectively. The mean cover crop biomass production was highest in hairy vetch (1.5 tons/ac), followed by Austrian pea (1.4 tons/ac), the mixture (1.1 tons/ac), and wheat (0.9 tons/ac), while the lowest biomass production was recorded in the crimson clover plots (0.2 tons/ac) (Hux et al., 2023). As expected, the biomass of legume cover crops demonstrated a lower CN ratio compared with wheat and the mixture. The CN ratio was lowest in hairy vetch (11.1), followed by Austrian pea (14.9), crimson clover (17.3), the mixture (38.8), and wheat (40.1). The simulated N uptake by cover crop biomass was highest for hairy vetch (272 lb/ac) and Austrian pea (201 lb/ac), followed by the mixture (59 lb/ac) and wheat (44 lb/ac), with the lowest uptake recorded for crimson clover (17 lb/ac).
Cover crops did not impact seed cotton yields in both the field experiment (DeLaune et al., 2020) and long-term simulations (Figure 2). This outcome is contrary to the conventional notion that factors such as sequestered N in cover crop biomass or soil water utilization by cover crops during winter fallow might limit cotton production. At this study location, Singh et al. (2022) also conducted simulations involving no-till with wheat and a mixture as cover crops under irrigated conditions, applying an equal amount of water for both cover and no-cover-crop treatments. Interestingly, they found that cotton exhibited lower N uptake under cover crop treatments, particularly during the initial cotton growth period; however, cotton yields were comparable between the cover crop and no-cover-crop treatments. Other field studies (e.g., Baughman et al., 2007; Lewis et al., 2018) within the SGP region found a decline in cotton yield following cover crop implementation due to increased moisture deficit at planting. In the TRP region, a lack of significant difference in dryland cotton yield between regenerative and conventional practices stands out as a noteworthy and positive outcome, aligning well with observations from center-pivot (DeLaune et al., 2020) and sub-surface-drip (DeLaune et al., 2012) irrigated cover crop field experiments.
Regenerative Practices and Soil Carbon Sequestration
The effect of regenerative practices on soil carbon sequestration is complex, site-specific, and strongly dependent on various biophysical conditions, including weather, soil type, and management interventions such as tillage system, cover crop species, and duration of adoption. The rate of carbon sequestration is driven by both the quantity and quality of plant biomass inputs. In the field experiment, legumes such as hairy vetch and Austrian pea were found to produce more biomass compared to wheat and crimson clover.
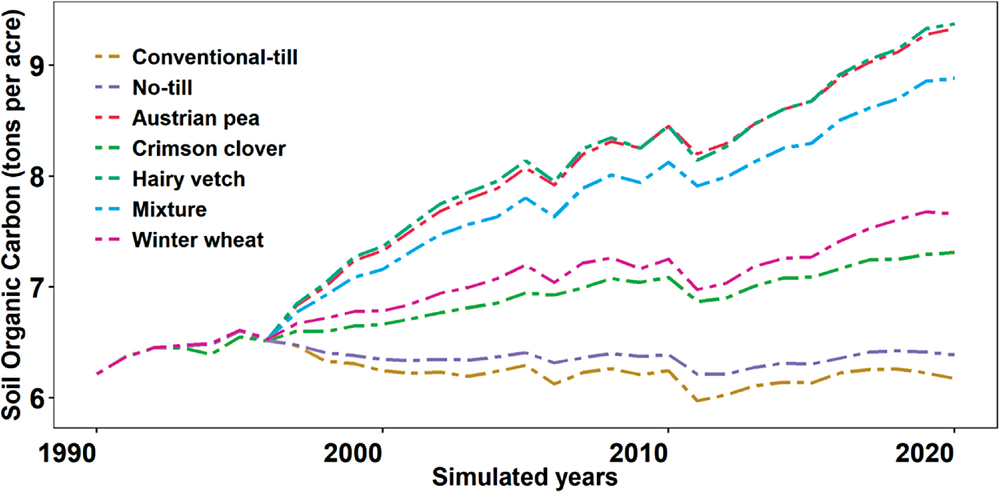
In this modeling study, cover crops in a no-till system exhibited a greater potential for soil carbon sequestration compared with systems without cover crops (Figure 3). The simulated carbon sequestration trend aligned with plant biomass trends, as returned residues played a crucial role in the system, particularly given that cotton yields were similar among treatments. Long-term simulations over a 25-year period indicated that hairy vetch and Austrian pea contributed the highest soil organic carbon stocks at a 0- to 12-inch depth, with a carbon accumulation rate of 230 lb/ac/year. Following closely were grass and legume mixtures with a carbon sequestration rate of 191 lb/ac/year. Wheat and crimson clover were estimated to sequester carbon at rates of 93 and 65 lb/ac/year, respectively. In contrast, no-cover treatments experienced carbon loss at rates of 9 and 26 lb/ac/year in no-till and conventional till systems, respectively. This suggests that carbon inputs from cotton alone are inadequate for soil organic carbon accumulation under no-cover-crop systems.
Studies reporting carbon measurements are limited in the SGP cotton production systems. In an irrigated cotton field experiment at Lamesa in the Texas Southern High Plains, greater soil organic carbon concentration was reported under no-till with rye cover crops, particularly in the upper 8 inches of the soil profile, compared with conventional tillage after 17 years of management (Lewis et al., 2018). However, soil organic carbon concentration was not significantly different in deeper subsurface layers (up to a 3-ft depth). Another study by Singh et al. (2022) found that planting rye, wheat, or mixed-species cover crops with a no-till system could lead to 11–19% more soil organic carbon accrual compared with no-cover crops in the 0- to 12-inch depth after 25 years under an irrigated system in the TRP.
What Is the Appropriate Time for Terminating Cover Crops?
In dryland systems, the primary focus is on maximizing cash crop productivity, making it essential to terminate cover crops at a time that balances biomass production benefits with the potential risks of excessive water use. When precipitation levels are below normal, producers are advised to adjust cover crop termination timing based on forecasted weather conditions and soil water status. In the Texas Rolling Plains (TRP) region, cover crops are typically terminated about six weeks before cotton planting.
In our simulation study, we evaluated the effects of five alternate termination dates: five, four, three, two, and one week before cotton planting.
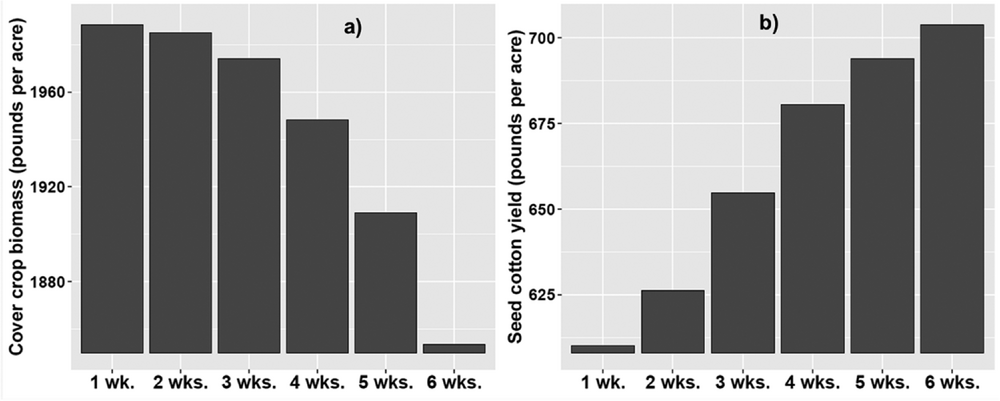
As expected, delaying cover crop termination significantly increased biomass production (Figure 4a), but at the cost of potentially reducing cotton yields (Figure 4b). In semi-arid regions, cover crop biomass production is highly dependent on spring precipitation. Historical weather records (1990–2020) indicate that even a one-week delay in termination resulted in increased biomass, largely due to May precipitation exceeding four inches. Successful biomass production relies on several management factors, including timely planting, appropriate species selection, optimal seeding rates, proper planting depth, and effective termination methods. Additionally, environmental conditions such as soil moisture, temperature, and overall weather patterns play crucial roles in biomass accumulation.
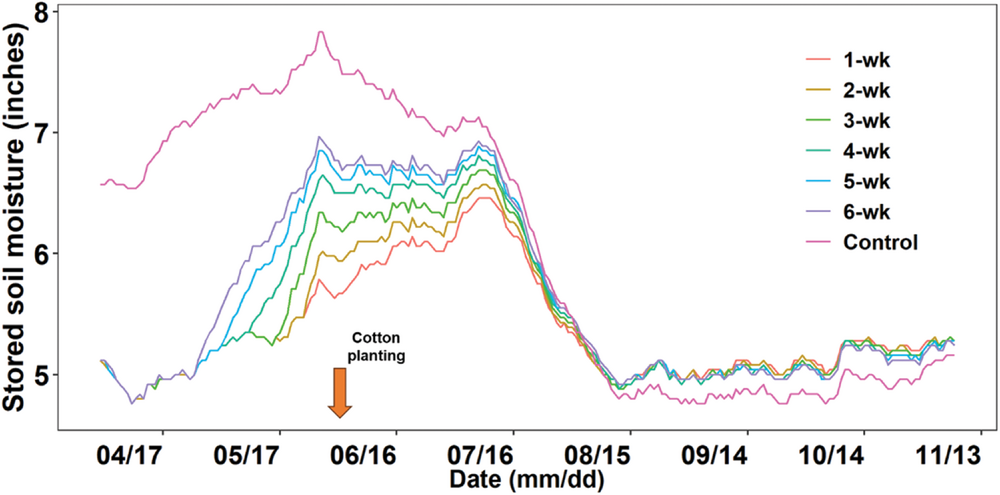
Cotton yields tend to decline with delayed cover crop termination due to soil water depletion during the cover crop growing season and inadequate spring precipitation to restore lost soil moisture. Comparative analysis showed that soil water levels were lower from April to mid-July in treatments with cover crops, compared to no-cover-crop conditions (Figure 5). Although May and June precipitation averaged eight inches, it was insufficient to fully recharge the soil profile. However, simulations indicated a net gain in stored soil moisture later in the cotton season under different cover crop termination dates compared to no-cover treatments. This gain was attributed to cover crop residues improving water infiltration, reducing runoff, and minimizing evaporation losses. Cover crop residues also mitigate wind erosion, prevent soil surface sealing during wet-dry cycles, and enhance soil structure and aggregation, further improving infiltration and reducing runoff.
A study by Burke et al. (2021) in the Southern Great Plains (SGP) similarly reported positive soil moisture retention during the cotton growing season under no-till management with a rye cover crop. In conclusion, for dryland cotton systems in the Texas Rolling Plains (TRP), the optimal time for terminating cover crops while avoiding yield penalties is approximately six weeks before cotton planting.
References
Baughman et al., 2007 – Baughman, T. A., Keeling, J. W., & Boman, R. K. (2007). On-farm conservation tillage programs to increase dryland cotton profitability (Rep. No. 05-643TX). Cotton Incorporated.
Burke et al., 2021 – Burke, J. A., Lewis, K. L., Ritchie, G. L., DeLaune, P. B., Keeling, J. W., Acosta-Martinez, V., Moore, J. M., & McLendon, T. (2021). Net positive soil water content following cover crops with no tillage in irrigated semi-arid cotton production. Soil and Tillage Research, 208, 104869.
DeLaune and Mubvumba, 2020 – DeLaune, P., & Mubvumba, P. (2020). Winter cover crop production and water use in Southern Great Plains cotton. Agronomy Journal, 112, 1943-1951.
DeLaune et al., 2020a – DeLaune, P., Mubvumba, P., Fan, Y., & Bevers, S. (2020a). Cover crop impact on irrigated cotton yield and net return in the Southern Great Plains. Agronomy Journal, 112, 1049-1056.
DeLaune et al., 2020b – DeLaune, P. B., Mubvumba, P., Fan, Y., & Bevers, S. (2020b). Agronomic and economic impacts of cover crops in Texas rolling plains cotton. Agrosystems, Geosciences & Environment, 3, e20027.
DeLaune et al., 2012 – DeLaune, P., Sij, J., Park, S., & Krutz, L. (2012). Cotton production as affected by irrigation level and transitioning tillage systems. Agronomy Journal, 104, 991-995.
Hux et al., 2023 – Hux, B. A., DeLaune, P. B., Schirmarcher, M. T., Gentry, T. J., & Mubvumba, P. (2023). Winter cover crop impact on soil health in Texas Rolling Plains dryland cotton. Agrosystems, Geosciences & Environment, 6, e20352.
Lewis et al., 2018 – Lewis, K. L., Burke, J. A., Keeling, W. S., McCallister, D. M., DeLaune, P. B., & Keeling, J. W. (2018). Soil benefits and yield limitations of cover crop use in Texas High Plains cotton. Agronomy Journal, 110, 1616-1623.
Singh et al., 2023 – Singh, J., Ale, S., DeLaune, P. B., & Barnes, E. M. (2023). Simulated effects of cover crops with no-tillage on soil and crop productivity in rainfed semi-arid cotton production systems. Soil and Tillage Research, 230, 105709.
Singh et al., 2022 – Singh, J., Ale, S., DeLaune, P. B., Himanshu, S. K., & Barnes, E. M. (2022). Modeling the impacts of cover crops and no-tillage on soil health and cotton yield in an irrigated cropping system of the Texas Rolling Plains. Field Crops Research, 287, 108661.
Self-Study CEU Quiz
Earn 1 CEU in Sustainability by taking the quiz for the article at https://web.sciencesocieties.org/Learning-Center/Courses. For your convenience, the quiz is printed below. The CEU can be purchased individually, or you can access as part of your Online Classroom Subscription.
- Water is the most limiting factor that can affect cotton production in a semi-arid climate.
- True.
- False.
- In this study, cover crops are planted in summer and terminated the next spring.
- True.
- False.
- What type of model is DNDC (DeNitrification-DeComposition)?
- Hydrologic model.
- Crop model.
- Biogeochemical model.
- Machine-learning model.
- Models are useful tools because
- they are inexpensive and complementary to field experiments.
- they are useful to assess the long-term impacts of changes in management.
- they are useful to run scenario analyses to answer various “what if” questions.
- All of the above.
- Which of the following statement is true about this study?
- Nitrogen was applied at pre-planting of cotton under the dryland experiment.
- Neither nitrogen nor irrigation water was applied to cotton or cover crop.
- Irrigation was applied with a center-pivot system.
- Irrigation was applied to cotton but not to cover crops.
- Both field experiment and modeling studies found a significant difference in seed cotton yield between treatments?
- True.
- False.
- In Figure 3, why do Austrian pea and hairy vetch plots show higher soil organic carbon stock than wheat plots?
- The quantity of biomass in Austrian pea and hairy vetch is lower than wheat.
- Wheat plots have lower biomass than Austrian pea and hairy vetch.
- The quality of biomass (i.e., carbon-to-nitrogen ratio) in Austrian pea and hairy vetch is higher than wheat.
- Both the quantity and quality of Austrian pea and hairy vetch biomass are higher than wheat.
- What factors can increase cover crop biomass production?
- Planting appropriate cover crop species within their optimal window.
- Seeding at optimum rates and managing planting depth.
- Using effective termination methods.
- All of the above.
- What is the appropriate time for terminating cover crops without yield penalty under dryland conditions in the Texas Rolling Plains?
- One week before cotton planting.
- Six weeks before cotton planting.
- Four weeks before cotton planting.
- Three weeks before cotton planting.
- In Figure 5, why is stored soil water at cotton planting less when cover crop termination is delayed?
- Precipitation was less.
- Cover crops used moisture for biomass accumulation.
- Cover crops terminated later had different soil types.
- Cover crop type was different in termination scenario.
Text © . The authors. CC BY-NC-ND 4.0. Except where otherwise noted, images are subject to copyright. Any reuse without express permission from the copyright owner is prohibited.