Crop selection and breeding and phosphorus cycling
A systems approach to making improvements on the farm and beyond the farm gate
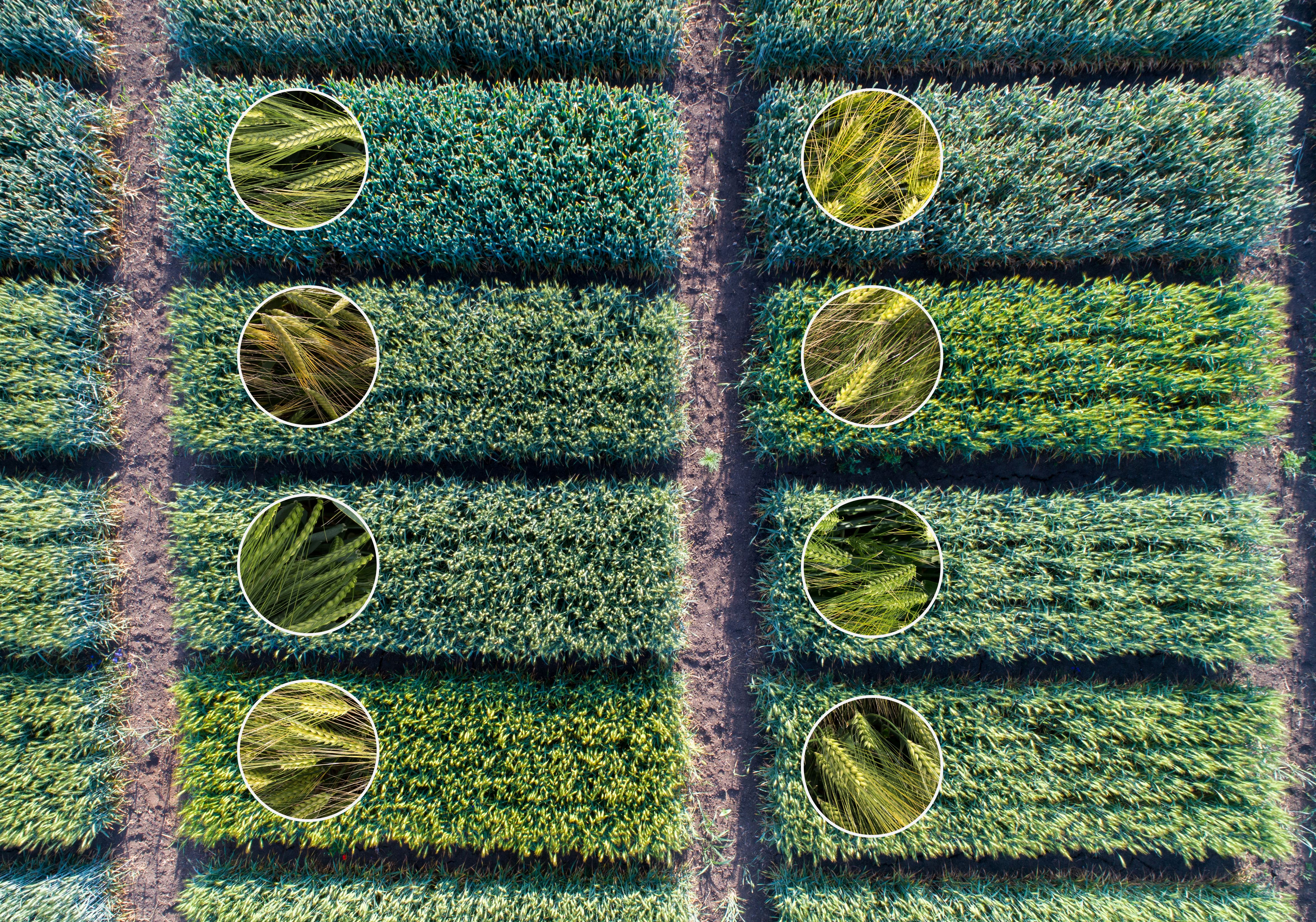
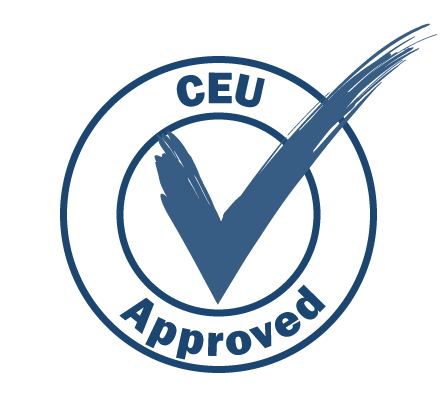
On-farm studies have reported low soil test phosphorus (P) status on organic farms. Low available P has been shown to decrease grain production in the long term and limits the productivity of legumes in commercial green manure crops. Greater phosphorus use efficiency would be beneficial to conventional farms as well. Crop selection and breeding for greater P use efficiency and P uptake under low soil test P has been proposed as a potential solution to tighten the P cycle on the farm. The authors of this article propose a hypothetical “catch and release” wheat ideotype with traits that facilitate enhanced P uptake under low-P supply and minimize off-farm harvest removal. Taken together, the proposed ideotype attempts to address P challenges on farms from a systems perspective, incorporating nutrient cycling, environmental considerations, and nutrition. Earn 1 CEU in Nutrient Management by taking the quiz for the article.
Phosphorus is an essential plant macronutrient, acting as a critical structural component of nucleic acids and playing a key role in energy transfer. Phosphorus (P) management is a particular challenge for organic farms on the Great Plains. While most conventional farming systems heavily rely on inputs of synthetic nitrogen (N) fertilizers, organic farms often maintain N levels through growing legumes within the green manure and forage phase of a crop rotation. However, replenishing P is more difficult on organic farms as crop harvest removal of grain or biomass continues to shrink the soil nutrient reservoir and manure is prohibitively expensive to transport for the size of the farms and proximity to a source.
On-farm studies have reported low soil test phosphorus status on organic farms (Knight et al., 2010). Low available P has been shown to decrease grain production in the long term and limits the productivity of legumes in commercial green manure crops. Greater phosphorus use efficiency would be beneficial to conventional farms as well as phosphate rock is a non-renewable resource and the over-application of phosphate-based fertilizers and manure has resulted in several algae bloom outbreaks in water bodies (Liu et al., 2021). Operating at a lower available soil P level to reduce off-farm losses, improving on-farm P uptake and use efficiency of crops, and reducing external P imports all play a key role in a farm’s productivity and sustainability.
Crop selection and breeding for greater P use efficiency (PUE) and P uptake under low soil test P has been proposed as a potential solution to tighten the P cycle on the farm (Rose et al., 2013). Phosphorus management on organic farms brings unique challenges as these farms rely heavily on biologically mediated nutrient supply, that is, mineralizing P from soil organic matter (SOM). Additionally, greater interest in legume cover crops and soil health is driving incorporation of biologically mediated nutrient supply management on conventional farms. Therefore, specific strategies and perspectives are required to optimize P uptake in partnership with crops and reduce off-farm P losses. The development of new crop cultivars that address P challenges on farms can contribute significantly to this goal.
Wheat ideotype to optimize phosphorus acquisition and utilization on organic farms
One approach to deploying genetic resources to achieve specific breeding goals is to develop a crop ideotype. An ideotype is defined as “a biological model that is expected to perform or behave in a predictable manner within a defined environment” (Donald, 1968). We propose a hypothetical wheat ideotype that can maximize P uptake and minimize off-farm P losses via grain P exportation for organic production systems (Figure 1).
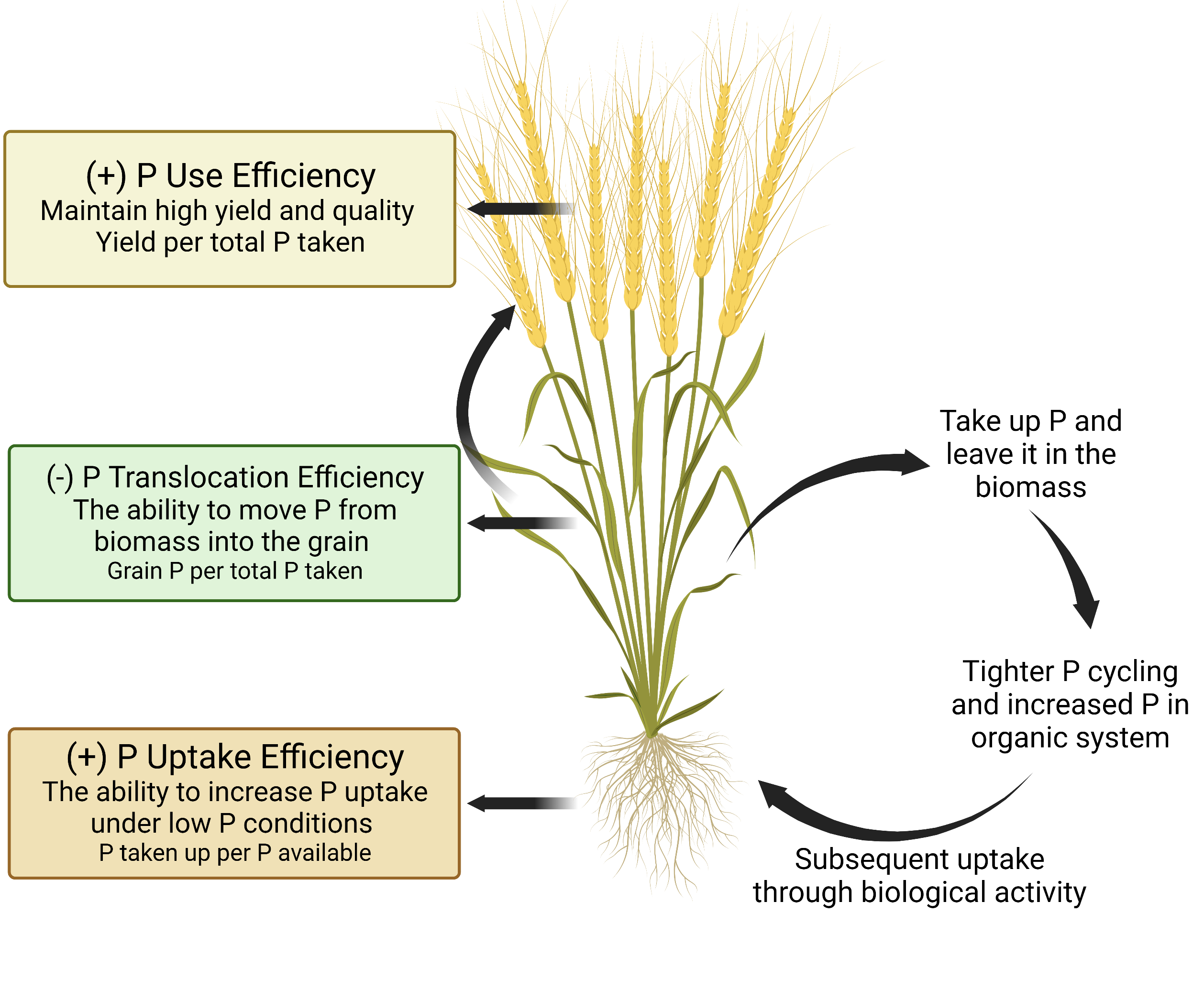
The P-efficient cultivar is characterized by three main features: (i) root-topsoil-foraging strategies to increase P acquisition, (ii) root-mining strategies to mineralize P from organic pools, and (iii) greater P utilization efficiency (e.g., greater yield per unit P applied). We also propose that reduced movement of P from shoot biomass into grain should be considered as an important feature for tighter P cycling to reduce off-farm export. While this concept is not new (Raboy, 2007; Rose et al., 2022), the importance of P translocation into grain relative to other traits has not been highlighted when considering overall PUE in addition to the potential of lower grain P as a food source beyond the farm gate and as a seed source. We wanted to explore the potential of incorporating plant traits to increase P acquisition and lower movement of shoot P into grain P by considering the distinctive nature and needs of agricultural production systems and the impact of lower grain P beyond the farm gate.
The phosphorus cycle in agroecosystems
The soil P cycle is a complex and dynamic process that involves a range of biological and geochemical transformations influenced by various environmental factors (e.g., soil moisture and temperature). Plants can only take up P in the form of HPO42– (soil pH 4.0–7.2) or H2PO4– (soil pH > 7.2), which are often referred to as plant-available P. Plant-available P concentration in the soil solution is typically low, less than 1% of the total P in the soil (Pierzynski, 1991).
The majority of soil indigenous plant-available P originates from weathering of minerals already in the soil. Once manure or P fertilizers are applied and P in soil exists as free ions, it can react with dissolved iron (Fe), aluminum (Al), manganese (Mn) in acid soils, or calcium (Ca) and magnesium (Mg) in alkaline soils to form phosphate precipitates (Figure 2). Plant-available P can also be adsorbed onto clays and the oxides of Al and Fe, taken up by plant roots, or incorporated into the organic-P pool as microbial infrastructure and/or organic matter (immobilization). Plant available P can be made available for plant uptake when microbes die, releasing P into the soil (mineralization). Through continuous biological and geochemical reactions, P available to plants and microorganisms are in a constant flux between mineralization/immobilization and adsorption/desorption processes.
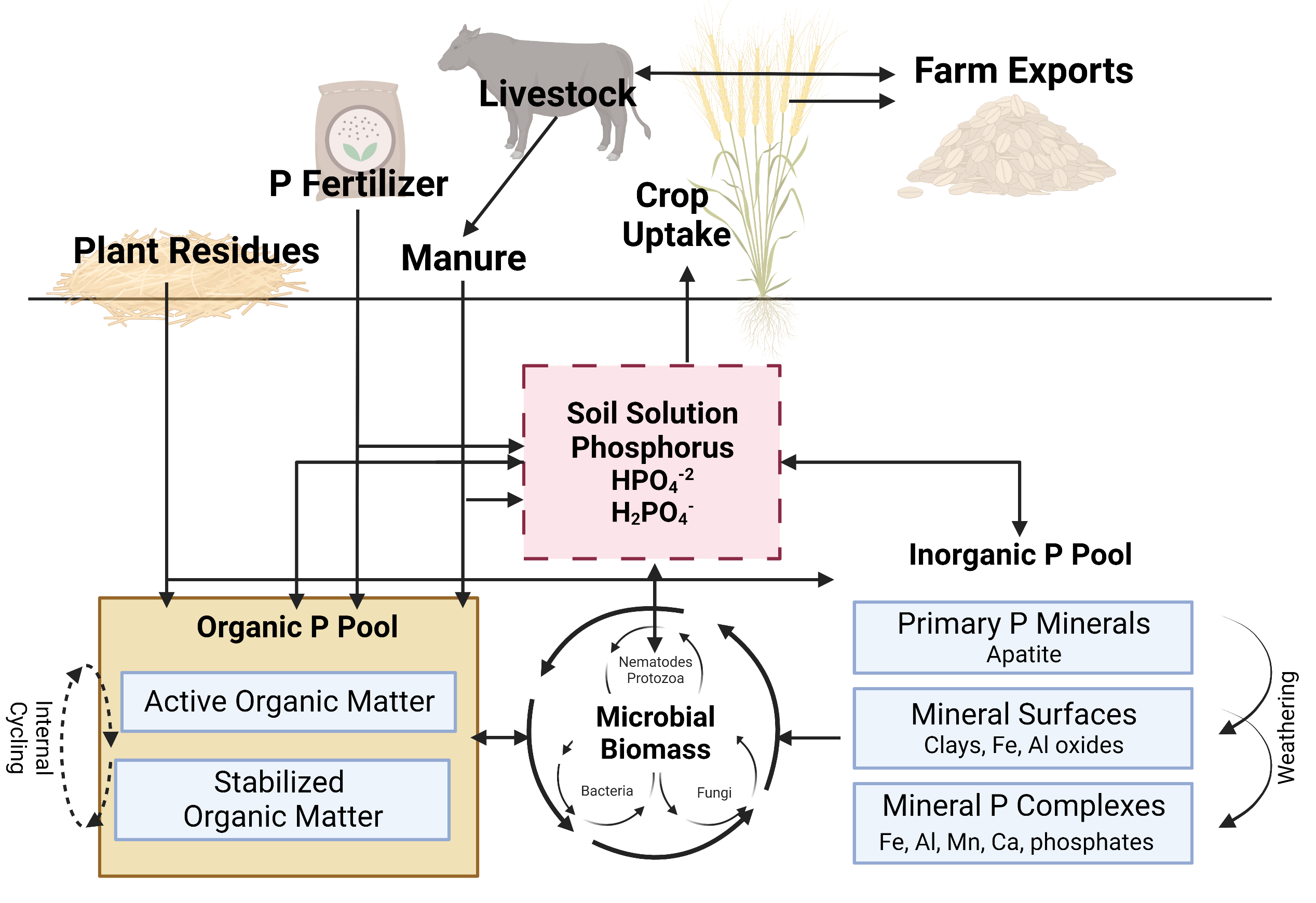
The organic phosphorus pool and microbial biomass phosphorus
Soil organic P is initially derived from animal wastes and plant residues and is synthesized by soil organisms. Many soil organic P compounds exist in the form of highly stable ring structures, making them resistant to mineralization and less accessible to plants.
It is estimated that organic P pools make up 30 to 80% of soil total P, depending on the cropping systems (Harrison, 1987). The microbial biomass component within SOM is the “live” fraction and responsible for mineralization of nutrients such as P. The abundance and activity of soil microbes are heavily reliant on C inputs as well as suitable soil moisture and temperature conditions.
Phosphorus uptake in plants and microorganisms
Phosphorus is relatively immobile in soil, meaning that plant roots and microorganisms must move towards P in the soil for uptake. When P in soil is taken up by plant roots or microorganisms, it creates a “depletion zone” adjacent to uptake site, necessitating constantly increased P access in the soil. There are two principal strategies to increase P access: (i) greater soil exploration to new zones of higher inorganic P (via better root growth or association with arbuscular mycorrhizal fungi) and (ii) P exploitation via chemical and biological P transformations to increase more available P uptake. Plants and microorganisms may use either strategies or a combination of both (Figure 3).
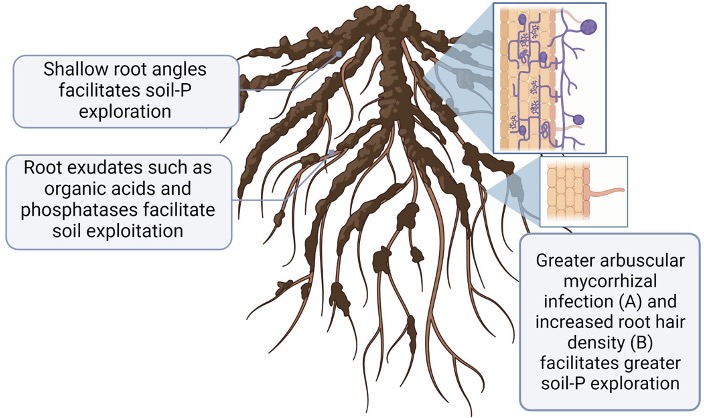
From the ground up: greater P uptake in organic soils
Increased physical exploration of soil
Greater exploration of the upper soil layer (0–10 cm) by crop root systems is essential due to the generally low P mobility in soils. The “topsoil-foraging” root architecture, which is characterized with wide basal and shallow seminal root angles, has been proposed to maximize soil exploration (Lynch, 2019). Structural characteristics such as greater root hair density and branching are important for P uptake as they increase root surface area and the volume of soil from which immobile P can be explored.
Association with arbuscular mycorrhizal fungi (AMF) is another strategy plants may use to increase soil exploration capacity. A mutualistic relationship between the host plant and AMF is characterised by a bi-directional nutrient transfer between AMF and the crop plant. The fungus receives carbon substrates from the host crop plant, and the plant receives nutrients in return.
Accessing where you are: Soil exploitation
Plants have multiple strategies that can increase soil P by making the less soluble P in the inorganic and organic P pools more available for plant uptake. One such strategy is through root exudation of organic acids into the rhizosphere to solubilize P from Fe and Al complexes in acidic soils and from Ca and Mg complexes in alkaline soils. Some plants can also excrete phosphatase enzymes into the rhizosphere to enhance mineralization by breaking down carbon and P ring structures within soil organic matter.
Organically managed soils are sometimes characterized by more abundant and diverse soil microbial communities (Mäder et al., 2002; Braman et al., 2016), which can lead to greater soil nutrient supply due to the increased mineralization capacity. However, relying only on the soil organic P pool can lead to organic matter mining and decomposition. Therefore, adequate crop residue return is essential on organic farms.
The consequences of low translocation of vegetative phosphorus to grain phosphorus
The phosphorus harvest index (PHI) is defined as the ratio of grain P to total plant P, and it represents the amount of P that has moved from the vegetative biomass into the grain during grain fill. While high protein content in wheat grain is valued in breeding programs, historically, greater grain P is not. Grain P is stored mainly as phytate. Phytate is poorly digested by monogastric mammals and often becomes a pollutant to water bodies from livestock and city wastes. Reducing grain P could contribute to decreasing off-farm P exportation.
Additionally, phytate is often considered an antinutritional compound as it strongly binds to micronutrients such as zinc (Zn) and iron (Fe). Low bioavailability of these minerals in cereal grains can lead to deficiencies in human populations who rely mainly on cereal foods for calorie intake. The phytate-to-Zn or phytate-to-Fe molar ratios in wheat grain have been generally used to categorize their bioavailability. Poor micronutrient bioavailability has implication for developing countries where people are at high risk of malnutrition. Breeding a wheat ideotype with high PUE and low grain phytate can potentially play an important role in alleviating the global prevalence of micronutrient deficiencies.
However, would reducing P translocation to grain adversely affect grain yield and quality? The relationships between PHI and yield are consistently weak, indicating that low P movement may not affect final grain yield. Currently, cereal crop breeding efforts are mainly focusing on increasing grain yield while maintaining protein. However, little is known about how decreasing seed P would play a role. Grain yield and protein are generally negatively correlated, so lower grain P in combination with lower yield would be expected to result in higher protein.
Will low grain P lead to a reduction in early season vigor due to depleted seed P reserves? Crop seedlings rely on P reserves for early growth and root establishment, up to three weeks after germination (Grant et al., 2001). Many studies have reported greater seedling vigor and increase P uptake due to faster root growth when comparing P-rich seeds with P-poor seeds (Rose et al., 2012). However, Julia et al., (2018) demonstrated that low-P seeds can access fertilizer P in as little as four days after germination. For organic production systems, May et al. (2022) reported that certain cover crops like black medic can increase soil-available P over time, which may result in greater seedling nutrition. Therefore, with the use of strategic ecological agronomy, there is a potential to select genotypes that can access and store greater P while translocating less P into the seed for better P cycling.
Crop residue potential to increase soil phosphorus availability
After grain harvest, organic farmers often incorporate the remaining crop residue into the soil either in the fall or early spring; this is typical for large, organic farms on the Great Plains where straw is not collected for animal bedding. Surface P leaching potential needs to be considered for no-till farms, especially in the spring when runoff is the highest. The ability of the ideotype to “release” P back into the soil system through mineralization processes may provide a valuable nutrient source for following crops and for building soil organic matter.
Implications for environmental protection and human nutrition
Phosphorus loss from agricultural lands poses a serious threat to water quality of Canadian watersheds. Over the last decade, major Canadian lakes such as Lake Winnipeg and Lake Erie have experienced severe algal bloom outbreaks (Liu et al., 2021). When excessive P from synthetic fertilizers or manure are applied to agricultural lands, they are subject to losses and being transported by surface runoff and drainage in variable proportions of dissolved P and particulate P (Hart et al., 2004). Improved P acquisition and utilization of the ideotype on an organic farm can contribute greatly to reducing P loss to water bodies by decreasing P inputs from organic sources. The proposed ideotype also addresses the concerns of producers and policymakers as it simultaneously reduces fertilizer/manure costs and environmental risks.
Conclusion
We propose a hypothetical “catch and release” wheat ideotype with traits that facilitate enhanced P uptake under low-P supply and minimize off-farm harvest removal. The ideotype would carry characteristics such as greater root exploration and exploitation strategies designed to interact with soil microbial communities.
Early seedling vigor is of particular importance to farmers because crops need to compete with early-season weed competition in cooler soils. Early-season weeds have the largest impact on final yield. Lower seed P may hinder early seedling vigor due to poorer seed nutrition, but this is unclear. Further research examining the impact of lower seed P, fertilizer placement, and genotypic effects on early vigor when the seed P reserves are low would be useful.
Lower seed P provides additional benefits of reducing exports off farm, thereby reducing P entering the wastewater system and polluting major water systems in North America. However, residue management needs to be considered to avoid P leaching from high P biomass on farm, especially in no-till production systems. Lastly, low seed P is beneficial from a nutritional standpoint as it leads to improved Zn and Fe bioavailability, potentially playing an important role in the nutritional portfolio of developing countries where Zn and Fe deficiency is prevalent. Taken together, our ideotype attempts to address P challenges on farms from a systems perspective, incorporating nutrient cycling, environmental considerations, and nutrition. As we move into a new paradigm of sustainable food production where external nutrients are becoming scarce and an increasing number of people face malnourishment, multi-pronged approaches will be required to address these challenges.
References
Braman, S., Tenuta, M., & Entz, M. H. (2016). Selected soil biological parameters measured in the 19th year of a long term organic-conventional comparison study in Canada. Agriculture, Ecosystems & Environment,233, 343–351. https://doi.org/10.1016/j.agee.2016.09.035
Donald, C. M. (1968). The breeding of crop ideotypes. Euphytica,17, 385–403. https://doi.org/10.1007/BF00056241
Grant, C. A., Flaten, D. N., Tomasiewicz, D. J., & Sheppard, S. C. (2001). The importance of early season phosphorus nutrition. Canadian Journal of Plant Science,81, 211–224. https://doi.org/10.4141/P00-093
Harrison, A. F. (1987). Soil organic phosphorus: a review of world literature. CAB International.
Hart, M. R., Quin, B. F., & Nguyen, M. L. (2004). Phosphorus runoff from agricultural land and direct fertilizer effects: A review. Journal of Environmental Quality,33, 1954–1972. https://doi.org/10.2134/jeq2004.1954
Julia, C. C., Rose, T. J., Pariasca-Tanaka, J., Jeong, K., Matsuda, T., & Wissuwa, M. (2018). Phosphorus uptake commences at the earliest stages of seedling development in rice. Journal of Experimental Botany,69, 5233–5240. https://doi.org/10.1093/jxb/ery267
Knight, J. D., Buhler, R., Leeson, J. Y., & Shirtliffe, S. (2010). Classification and fertility status of organically managed fields across Saskatchewan, Canada. Canadian Journal of Soil Science,90, 667–678.
Liu, J., Elliott, J. A., Wilson, H. F., Macrae, M. L., Baulch, H. M., & Lobb, D. A. (2021). Phosphorus runoff from Canadian agricultural land : A cross-region synthesis of edge-of-field results. Agricultural Water Management,255, 107030. https://doi.org/10.1016/j.agwat.2021.107030
Lynch, J. P. (2019). Root phenotypes for improved nutrient capture: an underexploited opportunity for global agriculture. New Phytologist,223, 548–564. https://doi.org/10.1111/nph.15738
Mäder, P., Fließbach, A., Dubois, D., Gunst, L., & Fried, P. (2002). Soil fertility and biodiversity in organic farming. Science,296, 1694–1697.
May, W. E., McConachie, R., & Entz, M. (2022). Self-regenerating black medic cover crop provides agronomic benefits at low nitrogen. Agronomy Journal,114, 2743–2761. https://doi.org/10.1002/agj2.21089
Pierzynski, G. M. (1991). The chemistry and mineralogy of phosphorus in excessively fertilized soils. Critical Reviews in Environmental Control,21, 265–295.
Raboy, V. (2007). The ABCs of low-phytate crops. Nature Biotechnology,25, 874–875.
Rose, T. J., Liu, L., & Wissuwa, M. (2013). Improving phosphorus efficiency in cereal crops: Is breeding for reduced grain phosphorus concentration part of the solution? Frontiers in Plant Science, 4. https://doi.org/10.3389/fpls.2013.00444
Rose, T. J., Pariasca-Tanaka, J., Rose, M. T., Mori, A., & Wissuwa, M. (2012). Seeds of doubt: Re-assessing the impact of grain P concentrations on seedling vigor. Journal of Plant Nutrition and Soil Science,175, 799–804. https://doi.org/10.1002/jpln.201200140
Rose, T. J., Thompson-Brewster, E., & Cornish, P. S. (2022). Phosphorus constraints to potential land area cropped under organic and regenerative systems in Australia. Crop and Pasture Science,73, 263–272. https://doi.org/10.1071/CP21578
Self-study CEU quiz
Earn 1 CEU in Nutrient Management by taking the quiz for the article at https://web.sciencesocieties.org/Learning-Center/Courses. For your convenience, the quiz is printed below. The CEU can be purchased individually, or you can access as part of your Online Classroom Subscription.
- Replenishing phosphorus (P) is more difficult on organic farms than conventional farms.
- True.
- False.
- What is an ideotype?
- A biological model which is expected to perform or behave in a predictable manner within a defined environment.
- The first example of a plant variety collected from a particular environment.
- An example of a plant variety that has the ideal physical appearance.
- A biological model that can perform or behave in a predictable manner in any environment.
- Which of the following is NOT a main feature of the P-efficient wheat cultivar proposed by the authors?
- Great P utilization efficiency.
- Root-topsoil-foraging strategies to increase P acquisition.
- Ability to take up P in any available form under any environmental conditions.
- Root-mining strategies to mineralize P from organic pools.
- What form is P available to plants in acidic soil?
- FePO₄.
- HPO₄²⁻.
- H₂PO₄⁻.
- AlPO₄.
- What in the soil is responsible for the mineralization of P?
- Microbes.
- Arbuscular mycorrhizal fungi.
- Mineral complexes.
- Animal wastes.
- What is a typical plant-available P concentration in soil solution?
- 10%
- 1–5%
- 2%
- Less than 1%
- Some plants can enhance mineralization through excretion of phosphatase enzymes that break down Fe, Al, Ca, and Mg complexes in soils.
- True.
- False.
- Which of the following characteristics of the roots are important for P uptake?
- Branching.
- Root hair density.
- Association with arbuscular mycorrhizal fungi.
- All of the above.
- Low bioavailability of ___ and ___, which strongly bind to phytate, in cereal grains can lead to nutritional deficiencies in humans.
- Ca and Mg
- Fe and Al
- Fe and Zn
- Zn and Mg
- What could be an off-farm consequence of reducing grain P?
- Increased eutrophication.
- Increased pollution.
- Reduced fertilizer costs.
- Decreased Zn bioavailability.
Text © . The authors. CC BY-NC-ND 4.0. Except where otherwise noted, images are subject to copyright. Any reuse without express permission from the copyright owner is prohibited.